Historically, materials and treatment options have limited the ability of dentist to treat diseased tissues. Traditional dentistry had no adequate therapeutic strategies for teeth with pulp necrosis, especially with incomplete root formation. These were subjected to apexification procedures or were extracted. However recently, the growing understanding of biological ideas about regeneration of dental tissues is revolutionizing the traditional dentistry at a fast pace. Regenerative approaches target to manage the diseased tissue beyond the traditional approaches that are based solely upon infection control [1]. Regenerative therapy promises number of clinical dental benefits, including biological strategies to repair teeth after carious damage, better treatment options of intraosseous periodontal defects and advanced grafting procedures for maxilla and mandible. Most research is directed toward regeneration of damaged dentin, pulp, resorbed root, periodontal regeneration and repair perforations. Whole tooth regeneration to replace the traditional dental implants is also in pipeline [2]. Additionally, a variety of tissue-engineering applications that will promote speedy healing of oral wounds and ulcers as well as the use of gene-transfer methods to manipulate salivary proteins and oral microbial colonisation patterns are feasible [3].
Regenerative Endodontics provides the hope of converting the non-vital tooth into vital once again. It focuses on substituting traumatized and pathological pulp with functional pulp tissue. This review article offers a detailed overview of present regenerative endodontic approaches aiming to replace pathological pulp tissues with healthy pulp tissue to revitalize teeth. It conjointly covers the basic trilogy elements of tissue engineering and also outlines the problems to be dealt before this emerging field contributes to clinical treatment protocols.
Materials and Methods
A web-based research on MEDLINE (www.pubmed.gov) was done for collecting data for the review. To limit our research to relevant articles, the search was filtered using terms Review, published in the last 10 years & Dental journals. Keywords used for research were “dental stem cells”(111 articles), “regenerative endodontics” (36 articles), “regenerative endodontic procedures” (17 articles), “Root Canal Revascularization” (9 articles), “Pulp Implantation” (8 articles). For every heading within the review, relevant articles were chosen and organized in order of publication date thus to follow topic closely. This review screened about 181 articles to get the desired knowledge update and only relevant information was compiled.
Discussion
The current (2012) American Association of Endodontists’ Glossary of Endodontic Terms defines regenerative endodontics as “biologically-based procedures designed to physiologically replace damaged tooth structures, including dentin and root structures, as well as cells of the pulp-dentin complex.”
Regenerative Endodontics relies on tissue engineering that provides a set of tools that can be used to perform regenerative therapy. The concept of tissue engineering was given by Charles Vacanti and Robert Langer from the Harvard Medical School. The first recorded use of the term tissue engineering was in an article entitled “Functional Organ Replacement: The New Technology of Tissue Engineering” by Prof. C. Vacanti published in 1991 in Surgical Technology International [4]. According to Langer and Vacanti (1993), tissue engineering is “an inter- disciplinary field that applies the principles of engineering and life sciences towards the development of biological substitutes that restore, maintain, or improve tissue function” [5].
Tissue engineering usually incorporates three key elements namely stem cells, scaffolds and growth factors.
Stem Cells/Progenitor Cells: Different populations of adult stem cells have been identified in the oral tissues. These include dental pulp stem cells (DPSCs),stem cells of the apical papilla (SCAP), inflammatory periapical progenitor cells (iPAPCs), periodontal ligament stem cells (PDLSCs), stem cells from human exfoliated deciduous teeth (SHED), dental follicle stem cells (DFSCs), tooth germ progenitor cells (TGPCs), oral epithelial stem cells (OESCs), gingival-derived mesenchymal stem cells (GMSCs), salivary gland stem cells (SGSCs), bone marrow stem cells (BMSCs) and periosteal-derived stem cells (PSCs) [6,7]. Although, stem cells have been identified in most oral tissues, the stem cells that are mostly involved in REPS include DPSCS, SHED, SCAP and PDLSCS. Certain studies have shown that non dental stem cells can also be stimulated for odontogenic response [8]. Cai et al., reported a possible method for growing teeth from stem cells obtained in urine [9]. In this study, pluripotent stem cells (iPSCs) derived from human urine were induced to generate tooth-like structures in a group of mice in the laboratory and reported success rates up to 30%. The generated teeth had physical properties similar to that of normal human teeth except hardness, which was about one-third the hardness of human teeth.
Scaffolds or Extra Cellular Matrix: A paediatric orthopedic surgeon W.T. Green, in the early 1970’s concluded through his experiments that with innovative biocompatible materials; it would be possible to generate new tissue by seeding viable cells onto scaffolds [10]. In the mid 1980’s, Dr Joseph Vacanti and Robert Langer designed and implemented scaffoldings synthetically fabricated in the laboratory that had physical and chemical properties that could be manipulated; they were biocompatible and biodegradable polymers as carriers of viable cells. Their original article describing the new technology was published in 1988 in Archives of Surgery [11]. Scaffolds provide a physicochemical and biological three-dimensional microenvironment for cell growth and differentiation, promoting cell adhesion and migration [12].
Types of scaffolds
Natural scaffolds: collagen and glycosaminoglycan.
Synthetic scaffolds: poly-L-lactic acid (PLLA), poly-glycolic acid (PGA), and their copolymers, poly-lactic-co-glycolic acid (PLGA).
Mineral scaffolds: hydroxyapatite and calcium phosphate.
Growth factors: They are extracellular secreted signals governing morphogenesis/organogenesis during epithelial mesenchymal interactions. Activity of common growth factors given in [Table/Fig-1].
Activity of common growth factors
Growth Factor | Activity |
---|
Bone morphogenetic proteins (BMP) | Bmp 4 from the epithelium induces the mesenchyme to be odontogenic.Bmp2, Bmp4, and Bmp7 from the enamel knot maintain the enamel knot and subsequent morphogenesis of epithelium. Also regulate the patterning of the tooth crown. Bmp 2, Bmp 4, Bmp 6, Bmp 7, and Bmp 11 are expressed during odontoblast differentiation. Bmp4 and Bmp 5 are expressed during ameloblast differentiation. |
Fibroblast growth factor(FGF), Platelet-derived growth factor (PDGF), Insulin-like growth factor-I or II (IGF), Colony stimulating factor (CSF), Epidermal growth factor (EGF) | Increase stem cell numbers |
Interleukins IL1 -13 | Modulate humoral and cellular immune responses |
Transforming growth factor-alpha (TGF-α) | Induces epithelial and tissue structure development |
Transforming growth factor-beta (TGF-β) | Promotes mineralization of pulp tissue |
Vascular endothelial growth factor (VEGF) | Regulates angiogenesis |
Regenerative approaches in endodontics
1. Root Canal Revascularization
This method involves a two- or multi-step procedure [13]. The first appointment is centered on proper access and disinfection of the root canal. After the tooth becomes asymptomatic, the second appointment focuses on removing the antimicrobial intracanal medicament, releasing various growth factors from dentin (e.g., by irrigating with ethylenediaminetetraacetic acid (EDTA)), delivering stem cells into the root canal by stimulating bleeding [14], creating a scaffold (e.g., blood clot or platelet-rich plasma) [15,16], sealing the tooth by placing a pulp space barrier (e.g., MTA or resin-modified glass-ionomer) and permanent coronal restoration to prevent bacterial re-infection [17]. Diagrammatic representation of the method is given in [Table/Fig-2].
Diagrammatic representation of root canal revascularization procedure
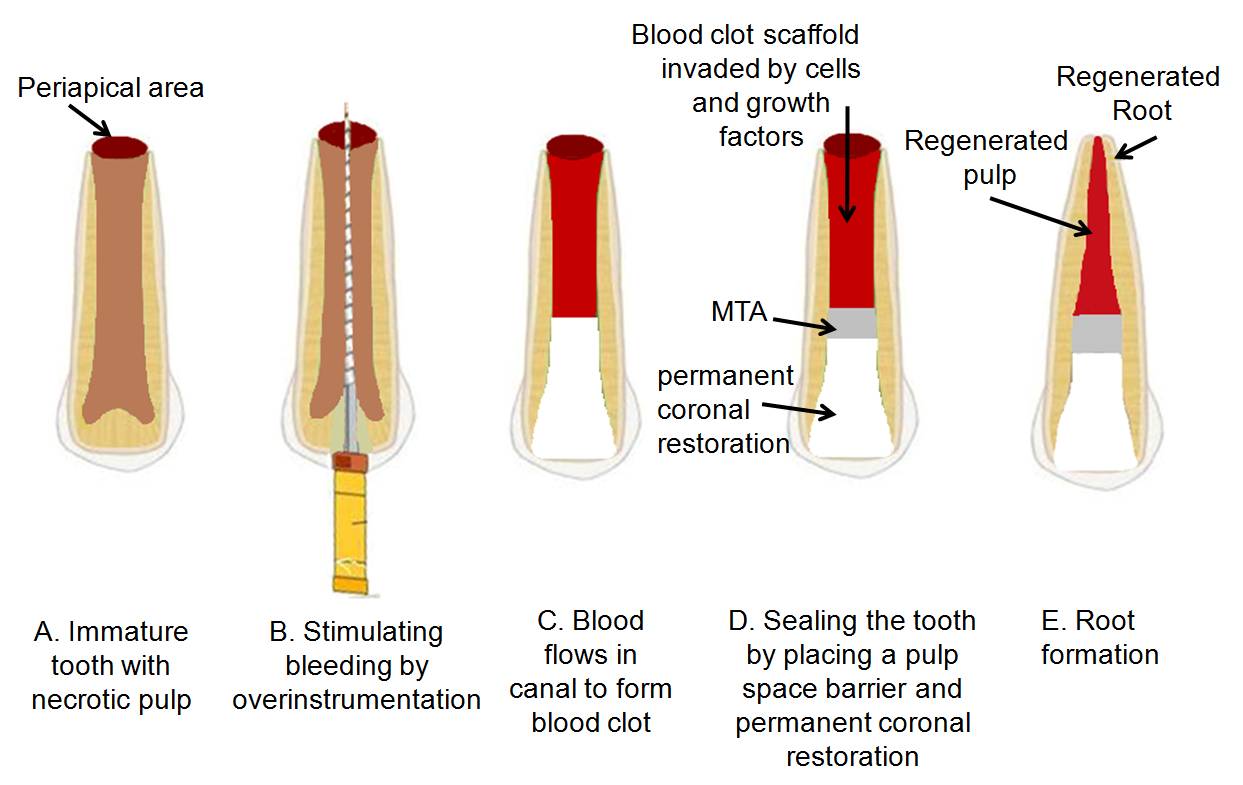
Normal, sterile granulation tissue formed within the root canal is assumed to assist in revascularization and stimulation of cementoblasts or the undifferentiated mesenchymal cells at the periapex, resulting in the deposition of a calcific material at the apex in addition on the lateral dentinal walls. The innovative idea of revascularization was introduced by Ostby and later, Rule & Winter documented root development and apical barrier formation in cases of pulpal necrosis in kids [18,19].
Several case reports have documented revascularization of necrotic root canal systems by disinfection followed by establishing bleeding into the canal system via over-instrumentation [15,19,20]. However, there have been reports of successful REPs without the formation of an intracanal blood clot [16]. Roots with odd shapes and blunted apices have also been reported [21, 22].
There are many advantages to revascularization approach that include simple approach, uses inexpensive armamentarium, avoid the likelihood of immune rejection as patient’s own blood cells are used and less microorganism transmission during replacement of the pulp with a tissue engineered construct [23].
However, the technique has its own drawbacks. The formation of a blood clot within the canal in these procedures can be challenging. Insufficient bleeding has been reported, particularly when epinephrine-containing local anaesthetics have been used. The second appointment, the use of local anaesthetic without a vasoconstrictor may better facilitate stimulation of apical bleeding [24].
The concentration and composition of cells trapped in the fibrin clot is unpredictable [23]. Enlargement of the apical papilla with a hand file or other sharp Instrument can damage the Hertwig’s epithelial root sheath (HERS) which plays a crucial role in dictating root development and shape.
2. Postnatal Stem Cell Therapy
Due to the restricted policies regarding ES cell research and possible immune rejections of human ES cells, researchers are now shifting attention on postnatal stem cells donated by the patients themselves or their close relatives for developing stem cell therapies. This method involves placing the postnatal stem cells into disinfected root canal systems for regenerative purposes. The application of postnatal stem cell therapy started in 1968, in which the first allogenic bone marrow transplant was successfully used in the treatment of severe combined immune deficiency [25]. Postnatal stem cells have been sourced from umbilical cord along with umbilical cord blood, peripheral blood, bone marrow, body fat, and various other body tissues, including the pulp tissue of teeth [26]. The postnatal stem cells for bioroot engineering as shown by Sonoyama et al., in a swine model if applied successfully in humans can provide future clinical approach to replace dental implants [27].
The disadvantages of postnatal stem cell method are low survival rates of injected cells, migration of cells to different locations within the body [28] possibly leading to aberrant patterns of mineralization and the hurdles of isolation and use of stem cell population for regenerative endodontic applications.
3. Pulp Implantation
In pulp implantation, replacement pulp tissue is transplanted into cleaned and shaped root canal systems. The source of pulp tissue may be a purified pulp stem cell line that is disease or pathogen-free, or is created from cells taken from a biopsy, that has been grown in various laboratories [29].
Dental pulp tissue engineering was first tested by Mooney’s groups [30]. Bohl et al., [31] reported that culturing pulp cells grown on polyglycolic acid (PGA) in vitro resulted in high cell density tissue similar to the native pulp. Buurma et al., [32] found that pulp cells seeded in PGA and implanted into the subcutaneous space of immunocompromised mice produced extracellular matrix. New blood vessels also infiltrated the cells/PGA implants invivo three weeks after the implantation.
The pulp stem cells must be organized into a three-dimensional structure that can support cell organization and vascularization. This can be accomplished by growing the pulp cells on biodegradable membrane filters of polymer nanofibers or on sheets of extracellular matrix proteins such as collagen I or fibronectin [33]. So far, growing dental pulp cells on collagen I and III has not been successful [34], but other matrices, including vitronectin and laminin, require investigation.
This technique has an advantage that it’s easy to grow cells on filters within the laboratory and collective sheets of cells are a lot more stable than unconnected cells administered by injection into empty root canal systems. Drawbacks of this method include requirement of specialized procedures to ensure proper adherence of cells to root canal walls, non vascular cell sheets and difficult to handle fragile filters.
4. Scaffold Implantation
A porous polymer scaffold can be seeded with pulp stem cells [35] and implanted into the empty root canal to create an engineered endodontic three-dimensional pulp tissue with similar cell organization and vascularization as of native pulp. A scaffold should contain growth factors to help in stem cell proliferation and differentiation, nutrients for promoting cell survival and growth, antibiotics to stop any microorganism in-growth within the canal, should exert mechanical and biological functions required by replacement tissue and should be biodegradable to avoid necessity of surgical removal [23]. However, it remains unsure which sort of scaffold material, collagen, polymer, or calcium phosphate, can offer the optimum survival substrate for DPSCs and PDLSCs. Food and Drug Administration (FDA) has recently approved collagen and polymer scaffolds for peripheral and cranial nerve repair, suggesting that these scaffolds might be approved by the FDA for dental repair in future.
Recent advances in the science have led to the development of synthetic polymer nanofibrous scaffolds that increases cell adhesion, differentiation and tissue formation by serving as a biomimetic extracellular matrix and have the capacity to form clinically relevant 3D tissue constructs. The three major fabrication techniques currently used to create nanofibrous scaffolds are molecular self-assembly, electrospinning and thermally induced phase separation [36].
Self-assembly generates small diameter nano-fibers in the lowest end of the range of natural extracellular matrix collagen whereas electrospinning generates large diameter nano-fibers on the upper end of the range of natural extracellular matrix collagen. Phase separation has generated nano-fibers in the same range as natural extracellular matrix collagen and allows for the design of macropore structures [37].
Bottino MC et al., [38] suggested that polymer-based antibiotic-containing electrospun scaffolds could function as a biologically safe antimicrobial drug delivery system for regenerative endodontics. Bottino MC et al., [39] also suggested electrospun nanocomposite fibrous material for Regenerative Endodontics with the potential to act like a scaffold and a drug-delivery device to aid in root maturogenesis and the regeneration of the pulp-dentine complex.
5. Injectable Scaffold Delivery
In this technique, engineered pulp tissue is administered in a soft three-dimensional scaffold matrix, such as chemical compound colloidal gel. Hydrogels are injectable scaffolds that can be delivered by syringe [40]. Research is being focused on making them photo-polymerizable so that they can form rigid structures once they are seeded into the tissue site. Photopolymerisable gels are promising tools for tissue engineering due to their high water content and tissue like elastic properties. The high degree of swelling due to their hydrophilic nature facilitates diffusion of oxygen and nutrients into the gel, making them suitable for tissue engineering scaffolds. Photopolymerisation reactions can occur at physiological temp and pH, resulting in the materials that can be gelled directly in the presence of cells or tissue thus feasible for in vivo formations [41].
Photopolymerisation can also be used to fabricate tissue-engineered scaffolds with micro-engineered capillary beds [42] by selectively exposing a light-sensitive solution to light. The exposed solution polymerizes whereas the unpolymerized masked solution gets washed away resulting in production of micro-channels [43] to provide passage for diffusion of oxygen and nutrients to support cells in tissue-engineered constructs.
6. Three-Dimensional Cell Printing
In this an ink-jet-like device is used to dispense layers of cells suspended in a hydrogel [44], to recreate the structure of the tooth pulp tissue. This technique could be used to precisely position various cells [45], and this methodology has the potential to create tissue constructs that mimic the natural tooth pulp tissue structure. The disadvantage of using the three-dimensional cell printing technique is that careful orientation of the pulp tissue construct according to its apical and coronal asymmetry would be needed throughout during placement into root canal systems.
7. Gene Therapy
In endodontics gene delivery method can be used to deliver mineralizing genes into pulp tissue to promote tissue mineralization. A carrier referred to as a vector is employed to introduce the therapeutic gene into the patient’s target cells. The vector can be given intravenously or injected directly into a particular tissue within the body, where it is taken up by the concerned target cells [46]. Or else, the patient’s sample cells are removed and exposed to the vector in a laboratory setting; the cells containing the vector are then reintroduced into the patient.
Virus that has been genetically modified to hold traditional human DNA is the most common type of vector used. Viral vectors studied by various researchers in gene therapy are given in [Table/Fig-3].
Various viral vectors studied
Viral vector |
---|
Retroviruses | Using retroviral vectors to treat X-linked severe combined immunodeficiency (X-SCID) represent the most successful application of gene therapy to date. |
Adenoviruses | The first gene therapy product to be licensed to treat cancer, Gendicine, is an adenovirus. Gendicine was approved by the Chinese food and drug regulators in 2003 for treatment of head and neck cancer. |
Adeno-associated virus (AAV) | There are six known human viral serotypes with most studies focusing on AAV-2. There is no known disease related with AAV, making it a suitable candidate for gene therapy. |
Lentiviruses | Long-term therapeutic efficacy of lentivirus-mediated gene transfer into the CNS has been reported in animal models of retinal photoreceptor regeneration, type VII mucopolysaccharidosis, Parkinson disease and metachromatic leukodystrophy. |
Herpes simplex virus type 1 (HSV-1) | It is currently the most extensively engineered for gene transfer. HSV vectors have been successfully used for treatment of animal models of cancer, PNS disease, certain brain diseases, spinal nerve injury and the treatment of pain. |
Non-viral options for gene delivery
Direct insertion of therapeutic DNA into target cells: simplest methodology.
Creation of an artificial lipid sphere (a liposome) with an aqueous core which carries the therapeutic DNA. It is capable of transporting the DNA through the membrane of the target cell. This delivery system is comparatively less effective than the other options.
Serious health hazards exist with the employment of gene therapy. These arise from the use of the vector (gene transfer) system, rather than the genes expressed. The FDA did approve research into gene therapy involving terminally ill humans; however approval was withdrawn in 2003 once a 9-year-old boy receiving gene therapy was found to have developed tumors in different parts of his body [47]. Attributable to the apparent high risk of health hazards, the future of gene therapy in endodontics is not very promising.
Hurdles to overcome for successful regenerative endodontics
Inspite of the impressive growth in regenerative endodontic field, there are various hurdles that must be overcome. They are outlined below.
Isolating, expanding and defining stem cell population for regenerative endodontic applications is a challenging task. The non-dental stem cells should also be explored for dental applications.
Appropriate vascularized scaffolds are required that are biodegradable and have the same rate of degradation as rate of formation of engineered tissue construct.
A better understanding and control over growth factors to get the desired quality tissue construct is required.
Better strategies of disinfection should be employed that does not interrupt the healing and integration of engineered pulp with the root canal walls and also reduce number of clinical sessions.
Methods to overcome certain procedural problems like insufficient bleeding at the second visit, tooth discoloration, lack of root development in the cervical area of the tooth that is critical for tooth strength should be found [48].
Unfavorable outcomes like poor or no root development, deposition of cementum-like hard tissue on root canal walls or formation of bony islands throughout the root canals [49] should be dealt with.
Current treatment approaches tend to stimulate more reparative than regenerative responses in respect of the new tissue generated [50].
Conclusion
Regenerative endodontic strategies have the potential to save lots of teeth which have compromised structural integrity. Each of the regenerative techniques has merits and demerits, and a few of the techniques are hypothetical, or at an early stage of development. As regenerative endodontics has clinical orientation, the success of the field relies on the final introduction of such therapies into clinical practice at large. The future will show which of the multiple approaches in regenerative endodontics will withstand the test of clinical usage.