Introduction
Advanced medical imaging modalities provide improved patient specific information which is pivotal in both diagnosis and treatment [1]. Further advancement of technology has made it possible to print/fabricate 3D models from the digital data. A 3D Printing (3DP), which originated in the late 1980s, is now being adopted in nearly every industry to create anything from customised eye wear to organs. Additive manufacturing is defined by “American Society for Testing Materials” internationally as “a process of joining materials to make objects from 3D model data, usually layer upon layer, as opposed to subtractive manufacturing methodologies” [2] Applications of 3D printing in medical and dental fields are vast [3]. Dentistry has a long association with CAD-CAM which involves subtractive manufacturing. Additive manufacturing overcomes the drawbacks of CAD. [4]. This paper reviews the 3D printing technology, its application in medical and dental fields and also briefly describes the revolution it can bring in other fields as well.
Origin and Progress of 3D Printing
The term “3D Printing” is relatively new but the technology is not all that new. In 1999, a patient with cleft palate was treated with the help of 3DP by Dawood A et al., [4]. The term “3D printing” was coined in 1995 by Ely Sachs at Massachusetts Institute of Technology, who authored a project of modifying an inkjet printer in such a way that a binding solution was extruded onto a bed of powder, like ink onto paper in inkjet printers. Charles Hull pioneered the modern idea of 3DP when he first described a vat polymerisation method, he founded the 3D systems corporation and a year later, released the SLA-1 in 1984 [5]. Medical professionals adopted 3D printing in the 1990s to produce engineered organs. The 2010s saw the emergence of various objects from cars to foods. Medical field advanced further by printing organs, tissues, prosthetic jaws and low-cost prosthesis [6].
Three-Dimensional Printing Process
The 3DP process has the following steps [4]:
Acquisition of 3D patient model, either physical model or digital model
Creating design STL file
3D Printing
Post processing
Acquisition of 3D Patient Model
Three dimensional models to be printed are made either with a Computer-Aided Design (CAD) package, via a 3D scanner, plain digital camera, photogrammetry software and x-ray or tomography images of medicine and dentistry [3]. Image sections are reconstructed with isotropic voxels of 1.25 mm or less. Model accuracy is due to thick section whereas extensive segmentation, STL refinement is required for very thin sections (<0.25 mm) [7].
Creating Design STL File
Three dimensional printers don’t support DICOM images which is standard image format for easy integration with server, scanners and printers, instead 3D printers accept file of an object with surface in a space. File format for these surfaces is Standard Tessellation Language (STL) and it is just a collection of triangles fit together in a puzzle forming object, necessary adjustment can be made in this file format and can be transferred to a 3D printer [7].
Three-Dimensional Printing
Today, there are at least 18 methods of 3DP and printing materials are also highly diversified [3]. Hence, selecting an appropriate 3D printer can be challenging. Since this technology is very helpful in medical and dental fields, its requirements should fall under the parameter for its use such as:
Cost effective
Easy to install
Colour capability
Time required to print
Biocompatibility
Acceptance of large number of materials
Should provide intended service
Less technique sensitive
Accept sterilisation
These factors should be kept in mind while choosing the printer and the importance to a specific work benefit should be given which will utilise the printer at its maximum capacitance [7].
Post Processing
An important step after an object has been printed, using most methods is post processing. Due to the layering involved in the processing, the printouts have rough surfaces [3]. Post processing of the printed model includes curing, polishing, cleaning and sterilisation, depending on the clinical usages [7].
Three-Dimensional Printing Systems
The 3DP technology used in medicine can be classified by technique, the material or the aimed deposition process used. There are seven technologies that are used for 3DP of which the first five is most commonly used in medicine. They include vat photopolymerisation, material jetting, binder jetting, material extrusion, powder bed fusion, sheet lamination and directed energy deposition [7,8]. The materials used for 3DP, to simulate various tissues, can also be classified as rigid, semi rigid, flexible and composite material [9].
I. VAT PHOTOPOLYMERISATION
This technology has three basic components: a high-intensity light source, a vat of photo-cured liquid resin, and a controlling system. Successive 2D layers are sequentially cured by exposing the liquid to a light source, such as laser that illuminates only the cross-section of the model. The resin solidifies on exposure and by successively lowering or raising the platform, each layer thickness is solidified until the final layer is complete, after which excess is removed and the model undergoes final curing in a UV chamber [5,7]. Vat photopolymerisation is frequently used, particularly for bone. Build platforms are wide ranging from sizes 12.5×12.5×12.5 cm to 210×70×80 cm [Table/Fig-1]. Dental models, dental implant guides and hearing aids are fabricated using smaller desktop devices [7].
Schematic diagram of vat photo polymerisation technology.
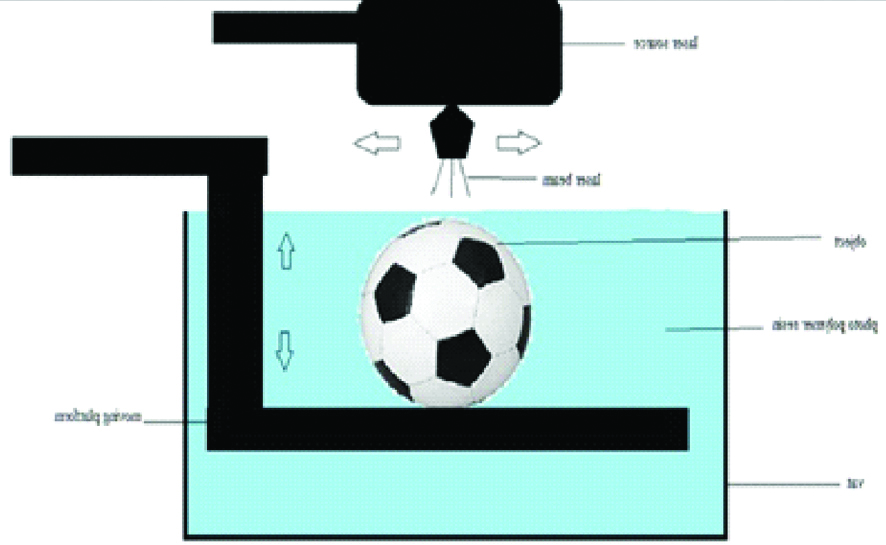
Many of the lithography-based approaches such as multiphoton polymerisation (2PP), Digital Light Processing (DLP), Stereo Lithography (SLA), poly jet and CLIP can be grouped into this category [1,10].
1. Stereolithography
Stereolithography is first type of 3D printer used and was developed in 1980’s and also first to be patented during that time. Laser beam was used to polymerise the photo polymer resin in two-dimension object just like our normal ink printers [11]. The basic principle is that a photosensitive monomer resin forms a polymer and it gets solidified when exposed to the light such as He-Cd laser beam [12]. This system consists of a bath, vat or container of photosensitive liquid resin, a model-building platform and an Ultraviolet (UV) laser, focussed by using computer-controlled mirror, for curing the resin [1,13]. After the layer is cured, subsequently another layer is added until the full model is complete [2,9,13,14].
The self-adhesive property of the materials causes the layer to bond to each other and eventually form a complete, en bloc 3D object. The unpolymerised liquid resin is removed by draining [11]. The model is then removed from the bath and cured for a further period in a UV cabinet to enhance the mechanical properties of the 3D objects [1,11,13-16]. The materials used for this technique must have photo curable moieties for photo cross linking which include acrylics and epoxides [Table/Fig-2]. Photo cross linkable Propylene Fumarate (PPF) is commonly used in SLA to fabricate 3D scaffolds. Newer materials such as Polycaprolactone (PCL), Poly Lactic Acid (PLA), Diethyl Fumarate (DEF) and Poly (trim ethylene carbonate) are being studied for tissue engineering applications [10,11,12,17].
Schematic diagram of stereolithography.
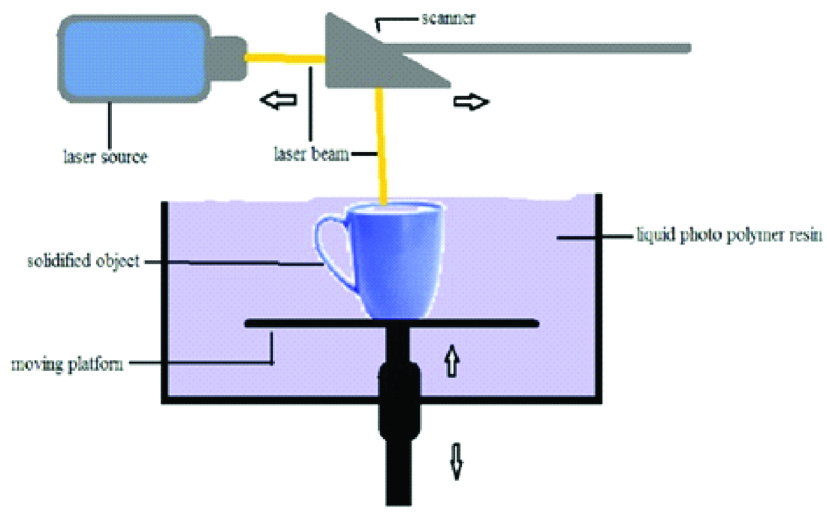
The advantages are that complex 3D objects can be fabricated with high accuracy, good surface finish, high mechanical strength and spatial resolution [10,12,17]. The disadvantages include scarcity of biocompatible resin, expensive equipment and materials, slower process, difficulty in removal of support structures, can be used only for polymers and post curing required.
2. Digital Light Processing
DLP is similar to SLA, both techniques utilise light to selectively cross-link photo resin in a layer by layer fashion to build free-standing objects [Table/Fig-3] [10,17]. The difference between DLP and SLA is that DLP uses a projector to cure an entire layer at a time. While DLP can be compared to stamping, SLA can be compared to drawing an object [13]. Digital Micro Mirror Device (DMD) is the heart of DLP projector and contains numerous tiny mirrors that have one off movement along two directions, thousands of times per second [13]. The utilised light sources have rapidly evolved from classical lamps to modern Light-Emitting Diodes (LED) covering a wavelength range from deep UV to visible light [10]. The materials that can be processed with DLP are non-filled photopolymers and slurries containing ceramic or metal particles.
Schematic diagram of digital light processing.

The advantages are best finish quality, good accuracy and mechanical strength and relatively fast process. The disadvantages of DLP are expensive materials and printing system, limited shelf and vat life and heat sterilisation needs to be done [2,13].
3. Photopolymer Jetting
The principle of polyjet is the same as the standard ink jet office printer, but in three dimensions [14]. It is performed by jetting state-of-the-art photopolymer through hundreds of nozzles on to the build platform. After spreading the droplets, the resin is cured using an ultraviolet lamp [1,9,14,18]. The building platform lowers and the process is repeated. A gel like support material is used/printed that surrounds the droplets, and can be easily removed manually or by stream of water [Table/Fig-4] [1,8]. Fully cured models can be handled and used immediately without any post-curing and post-processing procedures. Multiple types of materials can be jetted simultaneously and cured, thus fabrication of complex multi-materials objects is possible [19]. Layers as thin as 16 μm can be fabricated. Resins, waxes and silicon like rubber materials are used. The advantages include shorter and simpler post processing and mixing of different types of materials is possible. Wastage of materials and expensive technique are the disadvantages of the technique.
Schematic diagram of polyjet technology.
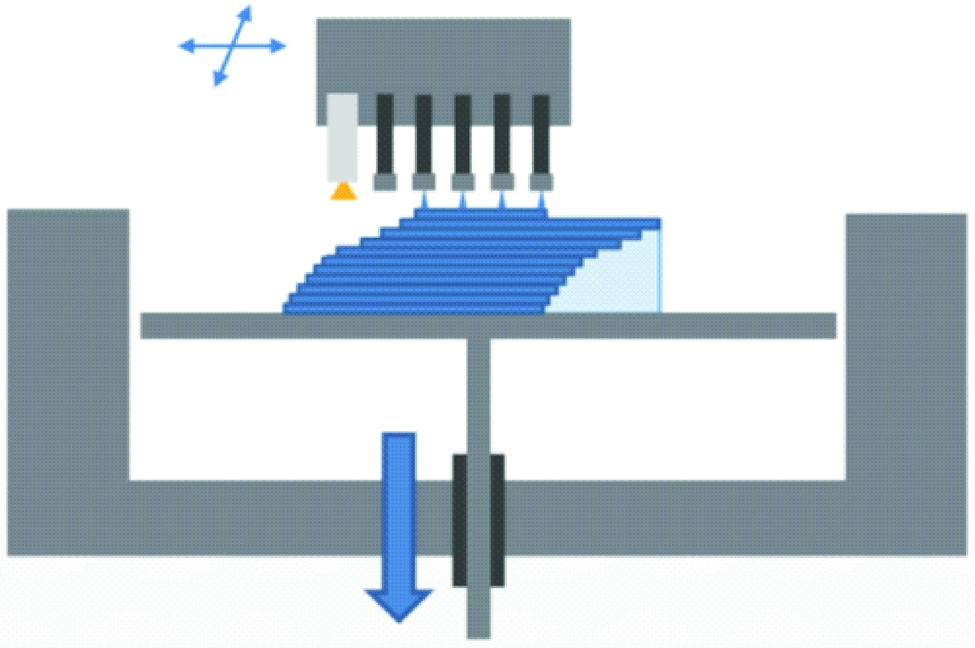
4. Continuous Liquid Interface Production (CLIP)
CLIP is a variety of vat photo polymerisation which uses an oxygen permeable film to inhibit polymerisation at the surface close to the UV source; it stops the formation of polymers in the liquid resin immediately above the window and prevents solidification in that region [Table/Fig-5] [10,19].
Schematic diagram of continous liquid interface production.
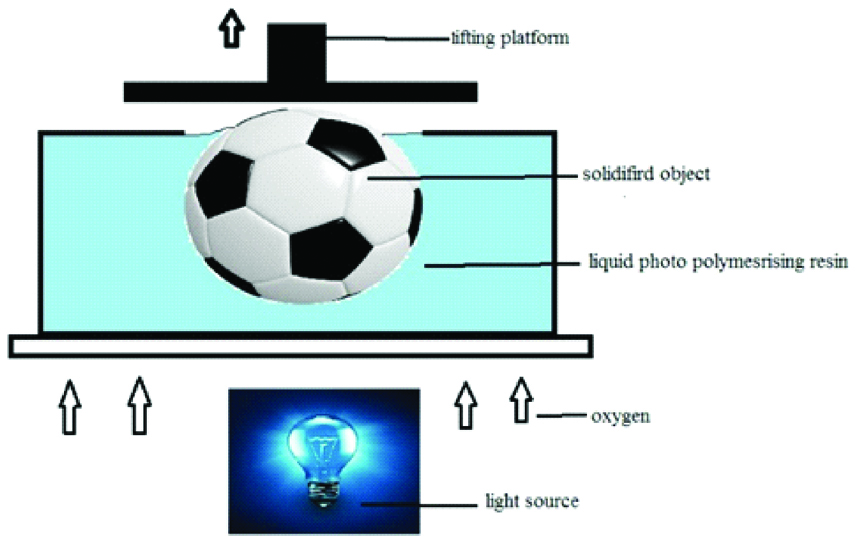
CLIP has been commercialised by the company Carbon 3D and has many advantages over other forms of SLA. The major advantages of CLIP are that it is faster than the traditional DLP [10].
5. Multiphoton/Two Photon Polymerisation (MPP/TPP)
Two photon polymerisation is also known as two photon absorbed photopolymerisation and two photon induced polymerisation, two photon lithography, two photon laser scanning lithography, or just direct laser writing. It is totally based upon the principle of different number of photons being used to start chemical reaction between molecules of monomer in a matrix [10,20].
Most currently used MPP systems are based on pulsed Ti: Sapphire fem to second lasers with pulse durations between 50-150fs [10,20]. The Charged Coupled Device (CCD) camera enables real time monitoring of the polymerisation process [Table/Fig-6] [20].
Schematic diagram of two photon polymerisation.
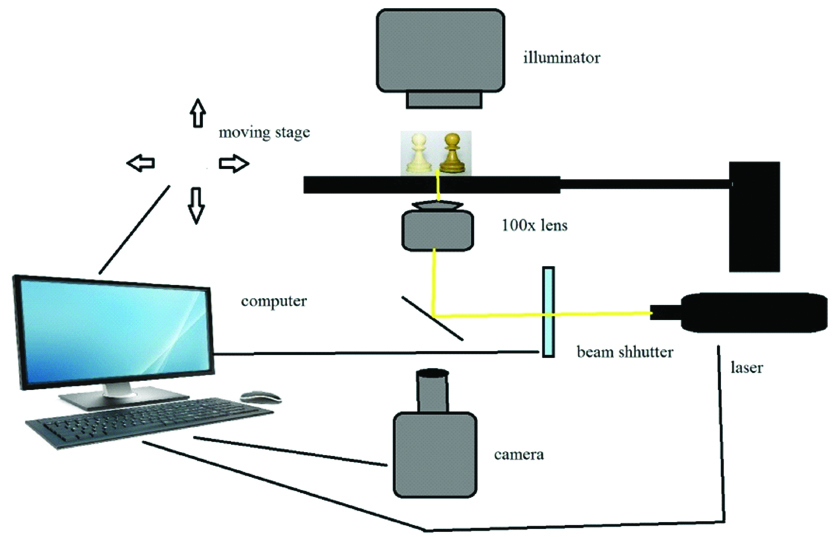
It has many applications in nanotechnology field where fabrication of small parts is done with precision, such as micro fluids or implant parts and many micro devices. It is most widely used in tissue engineering field and local drug delivery system where Nano and micro elements are used [21].
II. Material and Binder Jetting
This category of technology includes material jetting, aerosol jet printing and powder binding technology.
A. Material Jetting
This technique is called thermal inkjet printing and multijet printing. It is analogue to inkjet printing as these devices jet a liquid photopolymer onto a build tray and cures it with UV light [7]. When heat is used to cross link or to induce flow of the materials, the technique is called thermal ink jet printing [10,22]. The printers have two or more jetting heads which disperse an acrylic photopolymer (for the model) and wax (for support) simultaneously [1]. The build tray is incrementally lowered layer by layer [Table/Fig-7].
Schematic diagram of material and binder jetting technique.
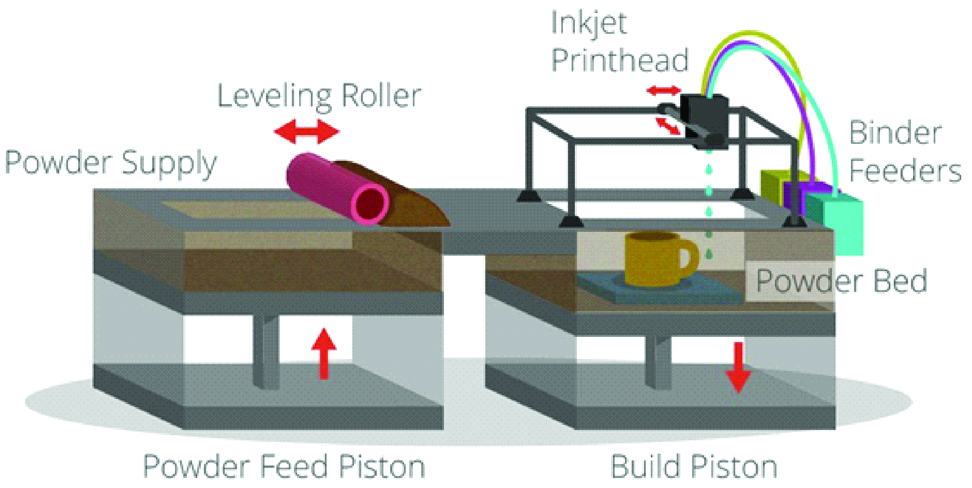
This technique also allows printing of clear models with internal structure such as nerves, teeth, tumours etc. Dental casts and dental implant guides are extensively printed using this technology [7]. The advantages include good resolution and minimal post processing. The disadvantage is expensive material.
B. Aerosol Jet Printing
The technique, also called mask less mesoscale materials deposition, was developed by OPTOMEC Inc. The process is based on atomisation of the building material which results in a dense aerosol which is transferred to the deposition head by an inert gas stream [Table/Fig-8]. Layer contours are printed by moving the building platform according to CAD data. The materials used in this technique include metals such as Ag, Cu, Ni, Al etc., poly (3,4–stipendiary theophany): poly (styrenesulforiate), polyvinylpyrrolidone, PMMA-based nanocomposite etc.
Schematic diagram of aerosol jet printing.
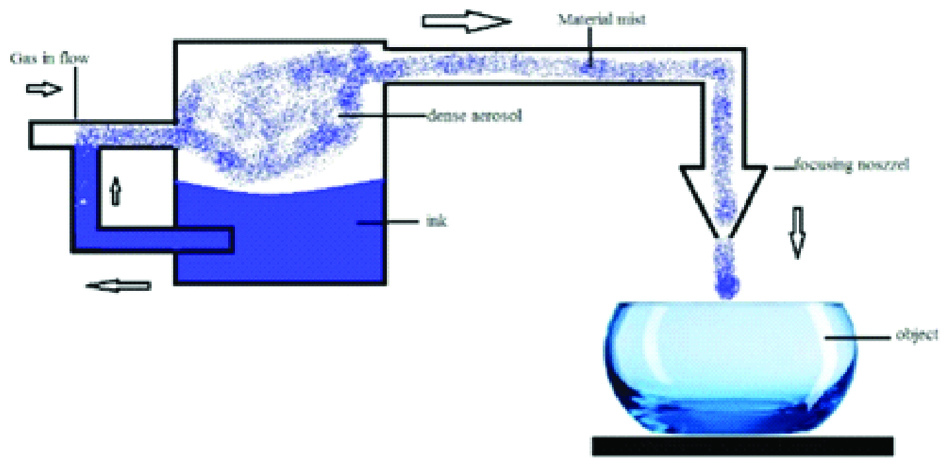
C. Powder Binding Technology
Also called Colour-Jet Printing (CJP) and Binder Jetting (BJ), this technique is similar to material jetting. In this technique, a powder layer is deposited then liquid binding agent is dispensed and finally the building platform moves downwards to enable printing of the next layer. After that the residual powder is removed with pressurised air [Table/Fig-9] [7,10]. As the prototype is being fabricated, no support structures are required, as the surrounding powder supports the unconnected parts [1]. The 3D fabricated object uses CMYK colouring ink cartridge for printing resulting in an identical range of colour. The powder used in this technique is starch, plastic cement, Polylactic Acid (PLA), poly (lactide-co-glycoside), PCL metallic ceramic powder. The binder solutions can be either aqueous or non-aqueous dispersion of inorganic materials like silica, aluminium nitrate, silver nitrate, or polymer solutions and dispersions which forms films upon drying and strongly adhere to the particles, thus bonding them together [10].
Schematic diagram of powder binding technology.
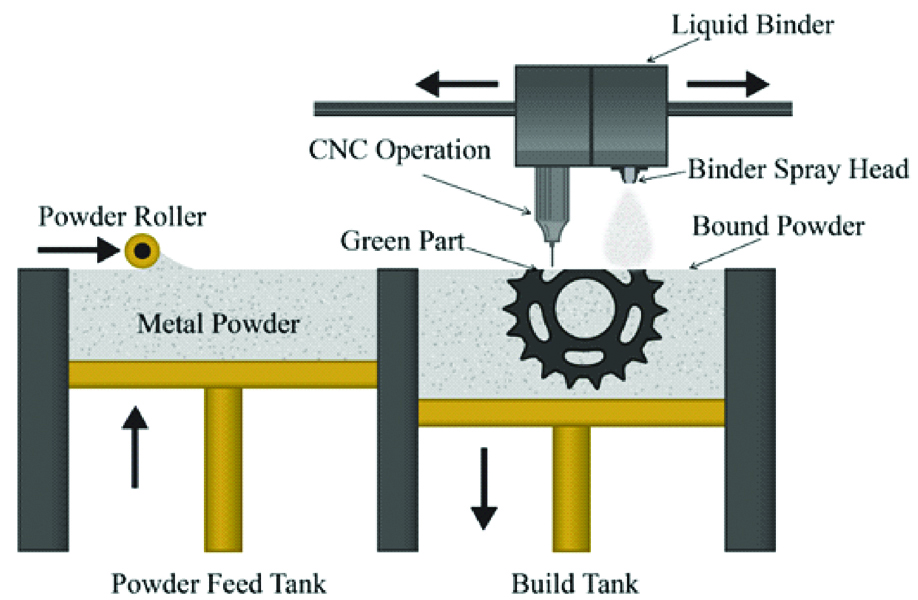
The advantages of this technique are that the materials are inexpensive, support structures are not required and the build platform is the largest [7].
III. Three-Dimensional Material Extrusion
Extrusion-based technology is the computer-controlled layer by layer deposition of molten and semi molten polymers pastes, polymer solutions and dispersions through a movable nozzle or orifice serving as the extrusion print head [Table/Fig-10]. It includes Fused Deposition Modelling (FDM) and 3D dispensing. FDM is limited to extrusion of thermoplastics at elevated temperature but 3D dispensing can use many other classes of materials like thermosets, rubbers, polyurethanes, silicones, organic and inorganic pastes, polymer latex, plastisols, biomaterial hydrogels, various functional polymers and biologically active ingredients and living cells. While in FDM, solidification of the polymer is achieved by polymer crystallisation and chain entanglement, 3D dispensing uses phenomena like reversible and irreversible cross-linking [10].
Schematic diagram of 3D material extrusion.
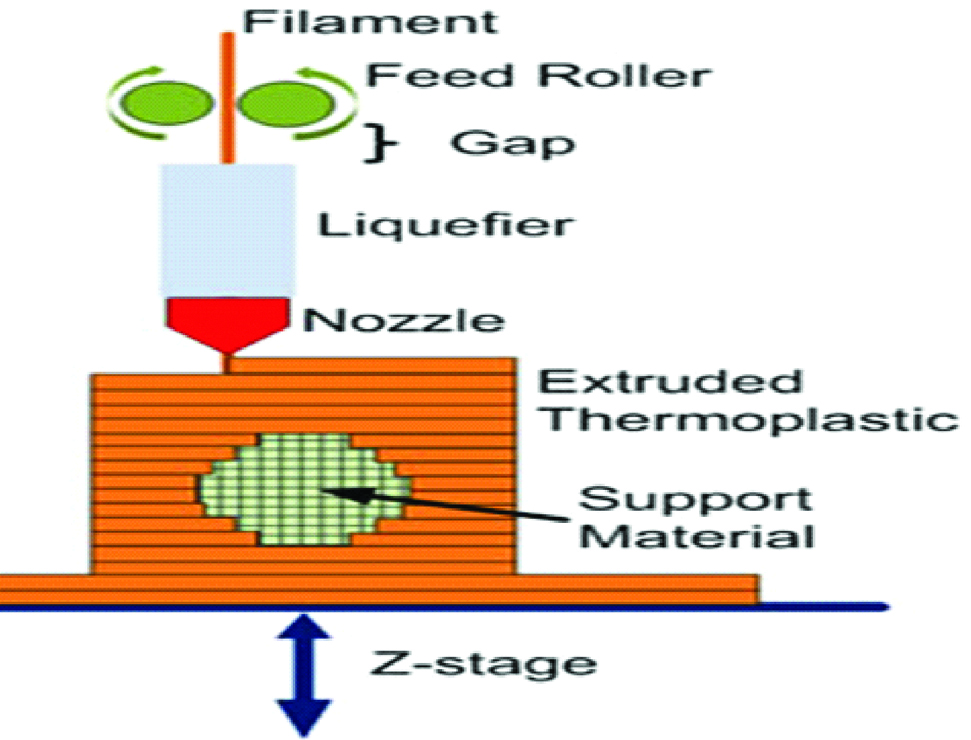
A. Fused Deposition Modelling (FDM)
It was developed by Scott Crump in 1988. Other terminologies used are “thermoplastic extrusion”, “plastic jet printing”, “fused filament method”, “Fused Filament Fabrication (FFF)”. This technology uses a continuous filament from a large coil which is extruded from a moving printing nozzle and deposited on growing work piece [23] [Table/Fig-11].
Schematic diagram of fused deposition modelling.
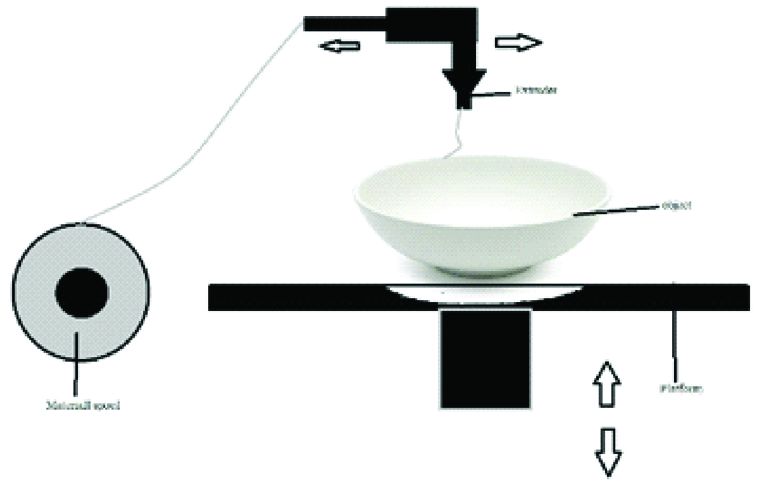
FDM uses a wide variety of materials that include synthetic polymers such as polyesters, natural polymers like alginate, polymer ceramic composition, Acrylonitrile Butadiene Styrene (ABS), Polylactic Acid (PLA), Polycarbonate (PC), Polyamide (PA), Polystyrene (PS), ligmin, rubber among many others [5,19,23].
The advantages include economical technique, ease of usage, mechanically and environmentally stable thermoplastics and good accuracy [1,7,11,23]. The disadvantages include poor surface finish and lack of finer details.
IV. Powder Bed Fusion Processes
This technology employs a fine resolution laser or electron beam to achieve binding of solid particles by partial or full melting. It uses powder bed and unlike vat photo polymerisation and material extrusion, it does not use photopolymers and does not require support [5,7]. This category includes Selective Laser Sintering (SLS), Direct Metal Laser Sintering (DMLS), Selective Laser Melting (SLM) and Electron Beam Melting (EBM).
A. Selective Laser Sintering
It was developed by University of Texas in 1989 by Carl Deckard and Joseph Beaman. The building procedure consists of powder deposition, powder solidification followed by the lowering of the build platform by one-layer thickness [Table/Fig-12]. These three steps are repeated until the final layer of manufactured part has been sintered [1,10,12,18,22].
Schematic diagram of selective laser sintering.
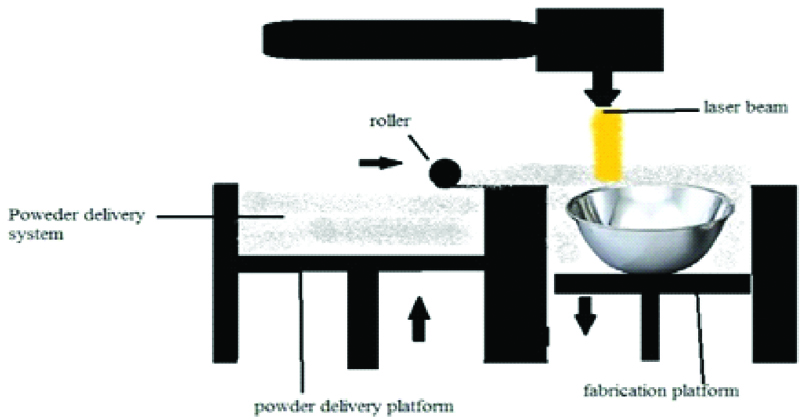
This technique uses a CO2 or Nd: YAG laser beam for scanning successive layers of powdered material to create a 3D object [8,24]. At the end of the manufacturing process, loose powder can be removed easily and reversed in successive runs [10]. The materials which can be used in SLS include nylon, polyether ether ketone, poly (e-caprolactone) (PCL), biphasic calcium phosphates, polymer/calcium phosphate ceramics, titanium alloys, wax, blend of poly vinyl alcohol and hydroxy path [2,5,11,13,15,20,24].
The advantages are the ability to use wide variety of materials and ability to print complex geometric structures and scaffolds. Expensive materials and equipment and rough finished surface are the disadvantages.
B. Direct Metal Laser Sintering
It was first developed by the EOS GMBH (Germany) company [1,25]. This technology is similar to SLS technology which prints plastic material and this technology prints metallic models/parts/components [18].
The DMLS process is performed by two different methods i.e., powder deposition and powder bed method. Due to the faster speed, the powder bed method is used frequently. There are two platforms inside the build chamber area, material dispensing platform and build platform. With the help of recoated blade, the material dispensing platform moves the powder to the build platform. The metal powder is fused into a solid part by melting it using laser energy 200 W high powered ytterbium fibre optic laser [1,26]. After a layer is built, the build piston lowers the build platform and the next layer of powder is applied [Table/Fig-13]. Materials used are chrome cobalt alloy with molybdenum, tungsten, silicon, cerium, iron, manganese and carbon as other additives [27]. The major advantages are: no support is required, no post-processing step and excellent printing quality. The disadvantages are: it is expensive and requires specific design guidelines [18].
Schematic diagram of direct metal laser sintering.
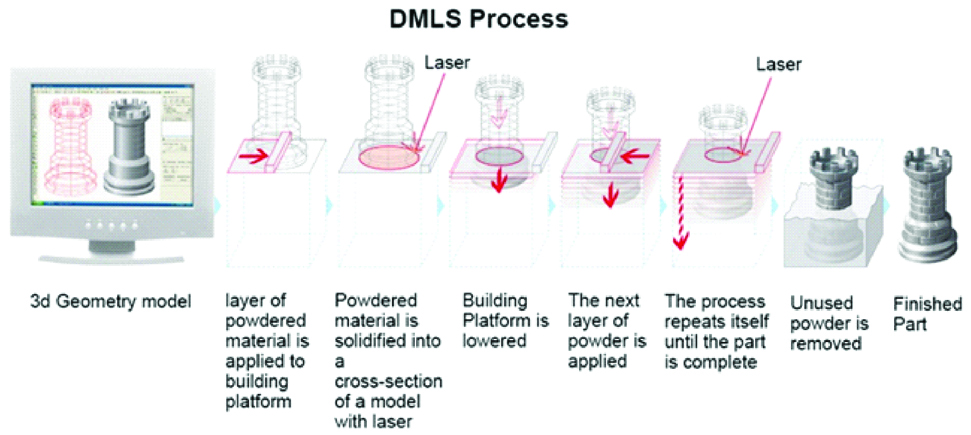
V. Sheet Lamination or Laminated Object Manufacturing
In the LOM process, thin sheets of paper, plastic or metal is stacked over one another, cut and pasted together to generate a 3D object [10,16,27]. Thus, LOM is a hybrid process of additive manufacturing and subtractive processing [10].
In the first step, adhesive-coated sheets are fed to the building platform and laminated using a heated roller. In the second step, the sheet is cut according to the contour provided by the 3D CAD data. Carbon dioxide laser or knives attached to the print head are used for contouring. New sheets are supplied by the rollers and the process is repeated until the object is completely built [10]. LOM process uses heat either on the build platform or on the roller in order to fuse the sheets together [16]. The materials used are paper; Polyvinyl Caprolactam (PVC) plastic or metal laminates [19].
VI. Direct Energy Deposition
It is an additive manufacturing technique which uses focussed thermal energy to create 3D objects. The high energy source could be an electron beam, a plasma welding torch or a laser. Energy is used to fuse materials like metal or powder by melting and then deposited layer by layer [8,10].
Applications of 3D Printing
3D printing can essentially produce anything from clothes to house and bridges, from tea cups to bikes and cars, from jewellery to food and also microbial colonies [8]. The different scopes under which 3D printing can be applied are industrial area, health care and well-being sector and consumer market.
3D printing is an established manufacturing method for past 30 years [28]. The industrial applications of 3DP include production of complex parts, prototypes, and to test a newly designed part or product before they are mass-produced [8,28]. Three-dimensional printing is already being used for the production of spare parts, customisation of products such as footwear, eye glasses etc. and for manufacturing complex devices [8,28]. Low cost personal 3D printers have entered the consumer market so that consumers can design products themselves, a development called marker movement. The most promising application of 3D printing is in the field of health care. 3D printing of surgical guides and models for surgical planning are the most reported cases in the literature. A study done by Van Assche N et al., evaluated the precision of transfer of a computer-based 3D planning, using re-formatted cone-beam images, for oral implant placement in partially edentulous jaws. The authors found that flat panel cone-beam CT images may be useful for computer-aided rapid prototyping of surgical guides for partial edentulism [29].
A study was undertaken by Chen J et al., to evaluate the mechanical and biomechanical performance of a novel custom-made dental implant fabricated by the selective laser melting technique with simulation and in-vitro experimental studies. They found that Selective laser melting 3D printing technology was able to recreate the customised implant designs with adequate dimensional accuracy and high density and strength [30].
Örtorp A et al., evaluated and compared the marginal and internal fit of three-unit FDPs in Co–Cr made using four fabrication techniques, and to conclude in which area the largest misfit is present using conventional Lost Wax method (LW), Milled Wax with lost-wax method (MW), Milled Co–Cr (MC), and Direct Laser Metal Sintering (DLMS). The result of the study stated that the best fit was found in the DLMS group followed by MW, LW and MC [31].
Xiong Y et al., evaluated the feasibility of using Three-Dimensional Printing (3DP) to fabricate porous titanium implants. Green, porous, titanium implants fabricated by 3DP was sintered under protective argon atmosphere at 1,200, 1,300, or 1,400°C. Uniform shrinkage and no obvious shape distortion after sintering was observed in Sintered implant prototypes. They found that feasibility of fabricating porous titanium implants by 3DP: pore size and pore interconnectivity were conductive to bone cell ingrowth for implant stabilisation. The mechanical properties matched well with those of the human bone [32].
Three-dimensional printing can be used in area such as oral surgery for making surgical guides and conducting various blocks to augment bone defects and for learning models to create mandibles and jaws. It is also used to restore the absence of parts of the external ear caused by congenital and acquired disorder and helps to print cartilage and blood cells [17].
Many removable appliances such as functional appliances, arch expansion appliances, retainer, arch wire, brackets, tray for indirect bonding can be made by 3DP which also helped to eliminate physical impression and put an end to bulky physical model storage [33].
In 2011, for the first time Belgium manufactured and implanted a full mandible directly from a 3D Printer without the need to create a mold. Models with pathology can be created from actual patients imaging data using 3D printing technique [34].
In 1994, Mankovich NJ et al., first applied 3D technology for skull reconstruction. Three-dimensional technology is also used to manage facial bone fracture such as orbital wall fractures and also used for the correction of syndromic craniosynostosis [35]. Recently, 3DP technology has been applied in fibular osteotomy and fixation. This helps to insert the dental implant in the ideal position [35].
Orthopaedics has the largest share in 3DP applications followed by maxillofacial surgery. The applications of 3DP in medical field can be categorised as follows:
Personal Treatment
a) Surgical planning and guidance tool
b) Implantable devices and prostheses
c) Bio printing-scaffolds, tissue engineering, hard organ printing, calcium phosphate scaffolds for bone regeneration
d) Drug delivery
e) Surgical reconstruction
Medical Research
a) Phantom fabrications
b) Microscopic bacterial communities
Medical Education and Training
a) Anatomic models
Orthopaedics has the largest share followed by maxillofacial surgery [36]. Three-Dimensional printed surgical guides are the widely published papers followed by models for surgical planning. The 3DP of surgical instrumentation and surgical guides have a great potential for the surgical planning. The surgical guides are produced using FDM or SLA out of sterile and bio-inert materials such as Acrylonitrile Butadiene Styrene (ABS), PMMA or polypropylene. In oral and maxillofacial surgery, 3D printed surgical guides are used for orthognathic surgeries, splints, osteotomies and contouring [25].
Anatomy based implants are created using 3D printing and have improved surgical performance. For hard tissues, metal implants have been used successfully in the jaws, hip and femoral bones, also in hemi-knee joint reconstruction [1]. Custom implants are mostly made of titanium, polyether ether ketone, epoxide acrylate hydroxyapatite, hydroxyapatite polymethyl methacrylate, polypropylene-polyester and non-specified acrylic based resin [28]. In complex cases, 3D Printing helps in producing custom implants, prostheses and also solves orthopaedics problem [22].
3D printing has become popular in biomedical research and tissue engineering due to its ability to replicate the intricate architecture as well as the cellular and constituent heterogeneity of tissues and organs [5]. The applications of 3D printing in tissue engineering include hard tissue scaffolds, construction of patient-specific tissues and hard organ printing [36]. Production of customised tissue and harvesting of autologous cells can be made possible by 3D printing [37]. Autologous stem cells such as adipose derived stem cells and induced pluripotent stem cells from patients can be used for organ printing. Multiple nozzle 3D printer has been employed to assemble the multiple autologous cells, growth factors and other biogenic agents [30]. 3D bio-printing can thus be used to create simple construct of cells, stem cells, tissues and even organs such as blood vessels, heart, skin, liver etc., [38,39]. Nearly 21 types of materials shave been used for bone tissue engineering but calcium phosphate ceramics are the most popular because of their excellent bio-activity, osteoconductivity and similarity in composition to bone [40].
The localised delivery of growth factors and drugs has attracted significant attraction due to the potential for dose reduction, controlled released pattern and negligible side effects compared to the systemic delivery. Three dimensional printed scaffolds have been used for this purpose [40]. Both high temperature and low temperature fabrication methods have been employed and each having its own benefits [5]. Drugs such as vancomycin, rifampicin, ofloxacin, tetracycline hydrochloride and growth factors such Vascular Endothelial Growth Factor (VEGF), bovine serum albumin, bone morphogenic protein have been studied [5,40].
Three-Dimensional printing is also being widely used for surgical reconstruction, medical research, fabrication of phantom, creating bacterial colonies and also anatomic models for medical education [1,7,41-44]. Personalised interventional treatment is made possible using 3D printing [1].
Limitation
The theoretical applications of 3DP in medicine are endless. Yet, there are barriers that need to be addressed so as to use this technology to its full potential. The barriers, limitations and challenges of 3D printing are as follows [12,37,42].
Cost and speed of production is the greatest limitation;
Development and standardisation of new materials;
Validation of the mechanical and thermal properties of existing materials and technologies;
Time spent in post processing affects the cost effectiveness of the model and reduces its usefulness in cases requiring urgent intervention;
Issues related to copyright;
Designers and engineer’s skill in 3D printer are limited;
Lower precision relative to other technologies.
Conclusion
Three-dimensional printing has a number of potential benefits and may play a significant part in the transition towards a more sustainable industrial system. It has been used successfully in many fields of medicine. But, the current limitations have restricted usage in specific circumstances. Hence, research in this field might open up new avenues enabling 3D printing to find application in everyday life.
[1]. Kim GB, Lee S, Kim H, Yang DH, Kim YH, Kyung YS, Three-dimensional printing: basic principles and applications in medicine and radiology Korean J Radiol 2016 17(2):182-97.10.3348/kjr.2016.17.2.18226957903 [Google Scholar] [CrossRef] [PubMed]
[2]. Chua CK, Leong KF, 3D Printing and Additive Manufacturing: Principles and Applications 4th edSingaporeWorld Scientific Publishing Co. Pvt. Ltd:26 [Google Scholar]
[3]. Dodziuk H, Applications of 3D printing in healthcare Kardiochirurgia i Torakochirurgia Polska 2016 13(3):283-93.10.5114/kitp.2016.6262527785150 [Google Scholar] [CrossRef] [PubMed]
[4]. Dawood A, Marti BM, Sauret-Jackson V, Darwood A, 3D printing in dentistry Brit Dent J 2015 219(11):521-29.10.1038/sj.bdj.2015.91426657435 [Google Scholar] [CrossRef] [PubMed]
[5]. Hull CW. inventor; UVP Inc, assignee. Apparatus for production of three-dimensional objects by stereolithography. United States patent US 4,575,330. 1986 Mar 11 [Google Scholar]
[6]. The history of 3D Printing: 3D Printing Technologies from the 80s to today. Available at: https: www.sculpteo.com/blog/2016/12/14/the-history of 3D Printing Technologies from the 80s to-today [Google Scholar]
[7]. Mitsouras D, Liacouras P, Imanzadeh A, Giannopoulos AA, Cai T, Kumamaru KK, Medical 3D printing for the radiologist Radio Graphics 2015 35(7):1965-88.10.1148/rg.201514032026562233 [Google Scholar] [CrossRef] [PubMed]
[8]. Van Wijk AJ, van Wijk I, 3D printing with biomaterials: Towards a sustainable and circular economy 2015 IOS pressAmsterdamDOI:10.3233/978-1-61499-486-2-i [Google Scholar]
[9]. Garcia J, Yang Z, Mongrain R, Leask RL, Lanchapelli K, 3D printing materials and their use in medical education: a review of current technology and trends for the future BMJ Stel 2018 4:27-40.10.1136/bmjstel-2017-00023429354281 [Google Scholar] [CrossRef] [PubMed]
[10]. Ligon SC, Liska R, Stampfl J, Gurr M, Mulhaupt R, Polymers for 3D printing and customized additive manufacturing Chem Rev 2017 117:10213-90.10.1021/acs.chemrev.7b0007428756658 [Google Scholar] [CrossRef] [PubMed]
[11]. Chia HN, Wu BM, Recent advances in 3D printing of biomaterials J Biol Engineering 2015 9(1):4DOI:10.1186/513036-015-001-410.1186/s13036-015-0001-425866560 [Google Scholar] [CrossRef] [PubMed]
[12]. Marro A, Bandukwala T, Mak W, Three-dimensional printing and medical imaging: A review of the methods and applications Curr Prob Diag Radiol 2016 45(1):2-9.10.1067/j.cpradiol.2015.07.00926298798 [Google Scholar] [CrossRef] [PubMed]
[13]. Azari A, Nikzad S, The evolution of rapid prototyping in dentistry: a review Rapid Prototyping J 2009 15(3):216-25.10.1108/13552540910961946 [Google Scholar] [CrossRef]
[14]. Groth CH, Kravitz ND, Jones PE, Graham JW, Redmond WR, Three-dimensional printing technology J Clin Orthod 2014 48(8):475-85. [Google Scholar]
[15]. Ibrahim AM, Jose RR, Rabie AM, Gerstle TL, Lee BT, Lin SJ, Three-dimensional printing in developing countries Plastic and Reconstructive Surgery Global Open 2015 3(7):3:e44310.1097/GOX.000000000000029826301132 [Google Scholar] [CrossRef] [PubMed]
[16]. Gross BC, Erkal JL, Lockwood SY, Chen C, Spence DM, Evaluation of 3D printing and its potential impact on biotechnology and the chemical sciences Anal Chem 2014 86(7):3240-53.10.1021/ac403397r24432804 [Google Scholar] [CrossRef] [PubMed]
[17]. Zaharia C, Gabor AG, Gavrilovici A, Stan AT, Idorasi L, Sinescu C, Digital dentistry—3D printing applications J Interdisciplinary Med 2017 2(1):50-53.10.1515/jim-2017-0032 [Google Scholar] [CrossRef]
[18]. Pîrjan A, Petrosanu DM, The impact of 3D printing technology on the society and economy J Information Systems & Operations Management 2013 7(2):360-70. [Google Scholar]
[19]. Tappa K, Jammalamadaka U, Novel biomaterials used in medical 3D printing techniques J Funct Biomater 2018 9:17doi: lo3390/jfb901001710.3390/jfb901001729414913 [Google Scholar] [CrossRef] [PubMed]
[20]. Peltola SM, Melchels FP, Grijpma DW, Kellomäki M, A review of rapid prototyping techniques for tissue engineering purposes Ann Med 2008 40(4):268-80.10.1080/0785389070188178818428020 [Google Scholar] [CrossRef] [PubMed]
[21]. Xing JF, Zheng ML, Duan XM, Two-photon polymerization microfabrication of hydrogels: an advanced 3D printing technology for tissue engineering and drug delivery Chem Soc Rev 2015 44(15):5031-39.10.1039/C5CS00278H25992492 [Google Scholar] [CrossRef] [PubMed]
[22]. Ventola CL, Medical applications for 3D printing: current and projected uses Pharmacy and Therapeutics 2014 39(10):704-11. [Google Scholar]
[23]. Mallesham P, Overview of fused deposition modelling process parameters Int J Modern Engineering Research www.ijmer.com>MECH>Version-3 [Google Scholar]
[24]. Shirazi SF, Gharehkhani S, Mehrali M, Yarmand H, Metselaar HS, Kadri NA, Osman N, A review on powder-based additive manufacturing for tissue engineering: selective laser sintering and inkjet 3D printing Science and Technology of Advanced Materials 2015 16(3):03350210.1088/1468-6996/16/3/03350227877783 [Google Scholar] [CrossRef] [PubMed]
[25]. Hoang D, Perrault D, Stevanovic M, Ghiassi A, Surgical applications of three-dimensional printing: a review of the current literature & how to get started Ann Transl Med 2016 4(23):45610.21037/atm.2016.12.1828090512 [Google Scholar] [CrossRef] [PubMed]
[26]. Venkatesh KV, Nandini VV, Direct metal laser sintering: a digitised metal casting technology J Indian Prosthodontic Soc 2013 13(4):389-92.10.1007/s13191-013-0256-824431766 [Google Scholar] [CrossRef] [PubMed]
[27]. Rengier F, Mehndiratta A, von Tengg-Kobligk H, Xechmann CM, Unterhinninghofen R, 3D printing based on imaging data: a review of medical applications Int J Comput Assist Radiol Surg 2010 5(4):335-41.10.1007/s11548-010-0476-x20467825 [Google Scholar] [CrossRef] [PubMed]
[28]. Okarma K, Fastowicz J, Quality assessment of 3D prints based on feature similarity metrics In International Conference on Image Processing and Communications 2016 Springer, Cham:104-111.10.1007/978-3-319-47274-4_12 [Google Scholar] [CrossRef]
[29]. Van Assche N, van Steenberghe D, Guerrero ME, Hirsch E, Schutyser F, Quirynen M, Jacobs R, Accuracy of implant placement based on pre-surgical planning of three-dimensional cone-beam images: a pilot study J Clin Periodontol 2007 34:816-21.10.1111/j.1600-051X.2007.01110.x17716317 [Google Scholar] [CrossRef] [PubMed]
[30]. Chen J, Zhang Z, Chen X, Zhang C, Zhang G, Xu Z, Design and manufacture of customized dental implants by using reverse engineering and selective laser melting technology J Prosthet Dent 2014 112:1088-95.10.1016/j.prosdent.2014.04.02624939253 [Google Scholar] [CrossRef] [PubMed]
[31]. Ortorp A, Jonsson D, Mouhsen A, Steyern PV, The fit of cobalt–chromium three-unit fixed dental prostheses fabricated with four different techniques: A comparative in vitro study Dent Mater 2010 27(4):356-63.10.1016/j.dental.2010.11.01521163516 [Google Scholar] [CrossRef] [PubMed]
[32]. Xiong Y, Qian C, Sun J, Fabrication of porous titanium implants by three-dimensional printing and sintering at different temperatures Dent Mater 2012 31(5):815-20.10.4012/dmj.2012-065 [Google Scholar] [CrossRef]
[33]. Mahamood S, Khader MA, Ali H, Applcation of 3D Printing in Orthodontics: A review IJSS 2016 3(11):267-70. [Google Scholar]
[34]. Choi JW, Kim N, Clinical application of three-dimensional printing technology in craniofacial plastic surgery APS 2015 42(3):267-77.10.5999/aps.2015.42.3.26726015880 [Google Scholar] [CrossRef] [PubMed]
[35]. Mankovich NJ, Samson D, Pratt W, Lew D, Beumer J, Surgical planning using three-dimensional imaging and computer modeling Otolaryngol Clin North Am 1994 27:875-89. [Google Scholar]
[36]. Tack P, Victor J, Gemmel P, Annemans L, 3D-printing techniques in a medical setting: a systematic literature review Biomedical engineering online 2016 15(1):11510.1186/s12938-016-0236-427769304 [Google Scholar] [CrossRef] [PubMed]
[37]. Wang X, Ao Q, Tian X, Fan J, Wei Y, Hou W, Tong H, Bai S, 3D bio printing technologies for hard tissue and organ engineering Materials 2016 9(10):80210.3390/ma910080228773924 [Google Scholar] [CrossRef] [PubMed]
[38]. Munaz A, Vadivelu RK, John JS, Borton M, Kamblen H, Nguyen NT, Three-dimensional printing of biological matters J Sci: Adv Mat Devices 2016 1(1):1-17.10.1016/j.jsamd.2016.04.001 [Google Scholar] [CrossRef]
[39]. Li J, Chen M, Fan X, Zhou H, Recent advances in bio printing techniques: approaches, applications and future prospects J Transl Med Epidemiol 2016 14:27110.1186/s12967-016-1028-027645770 [Google Scholar] [CrossRef] [PubMed]
[40]. Bose S, Vahabzadeh S, Bandyopadhyay A, Bone tissue engineering using 3D printing Materials today 2013 16(12):496-504.10.1016/j.mattod.2013.11.017 [Google Scholar] [CrossRef]
[41]. Connell JL, Ritschdorff ET, Whiteley M, Shear JB, 3D printing of microscopic bacterial communities Proc Nalt Acad Sci 2013 110(46):18380-85.10.1073/pnas.130972911024101503 [Google Scholar] [CrossRef] [PubMed]
[42]. Ford S, Despeisse M, Additive manufacturing and sustainability: an exploratory study of the advantages and challenges J Cleaner Prod 2016 137:1573-87.10.1016/j.jclepro.2016.04.150 [Google Scholar] [CrossRef]
[43]. Burman B, 3D printing: The new industrial revolution Business Horizons 2012 55:155-62.10.1016/j.bushor.2011.11.003 [Google Scholar] [CrossRef]
[44]. Parthasarathy J, 3D modeling, custom implants and its future perspectives in craniofacial surgery Annals of maxillofacial surgery 2014 4(1):910.4103/2231-0746.13306524987592 [Google Scholar] [CrossRef] [PubMed]