Thus, the present study was undertaken to find out the drug resistance mechanisms by production of β-lactamases as well as by biofilm formation in P. aeruginosa isolated from different clinical specimens of patients at a tertiary care hospital of Kolkata, West Bengal, India.
Materials and Methods
This cross-sectional prospective study was conducted in the Department of Microbiology, Medical College, Kolkata, West Bengal, India during July 2016 to June 2018, after the approval of Institutional Ethics Committee (MC/KOL/IEC/65/09-2015) and the informed consent was obtained from all the patients.
Sample Size
Sample size calculation was done using the formula n=z2pq/d2 where ‘p’ is the prevalence, q=1-p, d is the precision of the estimate. If the values are normally distributed, then 95% of the values will fall within 2 standard deviations of the mean and the value of z corresponding to 1.96. Based on a previously conducted study [9], the prevalence of MDR Pseudomonas spp. was approximately 50%. So, according to the calculation, 394 P. aeruginosa isolated from the samples received in the Department of Microbiology were included in the study. Infections with Gram-positive bacteria, samples of throat swabs and refusal of giving consent for taking samples were the exclusion criteria for this study.
Isolation and Identification of Pathogen
A total of 394 P. aeruginosa were isolated from different clinical samples (e.g., urine, blood, pus and wound swabs, sputum, ET suction materials etc.,) received from those patients admitted in hospital wards as well as Outpatient Department (OPD). Organisms were identified by Gram stain, cultural characteristics, colony morphology, motility and standard biochemical testing (oxidase and catalase production, carbohydrate fermentation, nitrate reduction, citrate and urease production) and species level identification was done by using VITEK-GN cards in VITEK-2 machine (bioMérieux India Private Limited). VITEK 2 system uses fluorescence-based technology for the identification and susceptibility testing of bacteria and is compliant with the Clinical and Laboratory Standard Institute (CLSI) guidelines 2018 [10].
Antimicrobial Susceptibility Testing
Antibiotic sensitivity test of isolated P. aeruginosa was performed in VITEK-2 system using AST-GN281 susceptibility cards in accordance with the CLSI recommendations [10]. Prevalence of MDR Pseudomonas spp. in the study was calculated according to the definition as non-susceptibility of the isolates to at least one agent in three or more antimicrobial categories.
Screening for ESBL, AmpC and MBL Production
Isolates resistant to CAZ (MIC ≥8 μg/mL) were screened for ESBL and AmpC β-lactamase production and the isolates resistant to MRP (MIC ≥8 μg/mL) were screened for MBL production. Accordingly, 181 (45.93%) isolates of P. aeruginosa screened for ESBL and AmpC and 115 (29.18%) isolates screened for carbapenemase production were further subjected to phenotypic confirmatory testing by disc antagonism test [11,12].
Phenotypic Detection of ESBL Production
Combined Disk Synergy Test (CDST) [11]
The test was performed by inoculating a Mueller-Hinton Agar (MHA) plate with the test strain. CAZ disks (30 μg) alone or with clavulanic acid (CAZ-CA/CAC) (30/10 μg) were placed on MHA plate. The agar plates were incubated at 37°C for 18 hour. An augmentation of ≥5 mm in the growth-inhibitory zone diameter of CAZ-CA (CAC) as compared to the zone diameter of CAZ disks was considered a positive result for ESBL production.
Phenotypic Detection of AmpC Production
Cefoxitin-Cloxacillin Double Disc Synergy test (CC-DDS) [12]
This test was based on the inhibitory effect of cloxacillin on AmpC production. The strains to be tested were inoculated on MHA using McFarland 0.5, followed by putting the discs containing 30 μg of Cefoxitin (CX) and 30 μg of CX plus 200 μg of cloxacillin disk (CXX) (Hi Media, Mumbai, India). with centres at least 24 mm apart and incubated at 37°C for 18-24 hour. A difference of ≥4 mm in the inhibition zones of CX-CXX and that of CX alone was considered as AmpC producer.
Phenotypic Detection of Carbapenemase (Metallo- β-Lactamase, MBL) Production
CDST by using EDTA
An amount of 186.1 gram of disodium EDTA.2H2O was dissolved in 1,000 mL of distilled water to prepare 0.5 M EDTA solution [13]. The pH was adjusted to 8.0 by using few drops of NaOH and was sterilised by autoclaving. A 0.5 McFarland inoculum of the organisms was spread on each MHA plate. Two 10 μg MRP disks were placed on MHA plate seeded with the test strain. Then, 10 μL of EDTA (750 μg) was added to one of the disks. After overnight incubation, a difference of ≥5 mm in zone diameter between the disks containing MRP plus EDTA solutions and that containing MRP alone was considered positive for MBL production.
Detection of Carbapenemase Encoding Genes among Screened Isolates
The presence of carbapenemase encoding genes was determined by multiplex PCR using primers (ReadyMade” Primers, Integrated DNA Technologies) targeting blaVIM, blaIMP, and blaNDM [Table/Fig-1] [14].
Primers used for Multiplex PCR for NDM, VIM, IMP carbapenemases.
PCR name | Targeted gene | Primer sequence (5′ to 3′) | Amplicon size |
---|
Carba | blaNDM | Forward | ACT TGG CCT TGC TGT CCT T | 603 bp |
Reverse | CAT TAG CCG CTG CAT TGA T |
blaVIM | Forward | TGT CCG TGA TGG TGA TGA G T | 437 bp |
Reverse | ATT CAG CCA GAT CGG CAT C |
blaIMP | Forward | ACA YGG YTT RGT DGT KCT TG | 387 bp |
Reverse | GGT TTA AYA AAR CAA CCA CC |
NDM: New Delhi metalo β-lactamase; VIM: Verona integron-encoded metallo-β-lactamase; IMP: Imipenemase
Bacterial cells were lysed using boiling method to obtain boiled template containing genomic DNA [15]. For amplification, 4 μL of template DNA was added to a 16 μL of 2x QIAGEN Multiplex PCR Master-Mix and RNAase free water was added. For amplification the cycle condition was initial denaturation at 95°C for 15 minutes, 94°C for 30 seconds annealing at 57°C for 90 seconds and elongation at 72°C for 90 seconds. The cycles were repeated 30 times and all primer sets had a final extension of 72°C for 10 minutes. Quality control was performed with each run using positive control strains of Klebsiella pneumoniae ATCC BAA2156 for NDM 1, Klebsiella pneumoniae NCTC 13440 for VIM, Escherichia coli NCTC13476 for IMP.
Biofilm Production by Tissue Culture Plate Assay
Biofilm production was done by tissue culture plate assay described by Christensen GD et al., [16]. Isolates which were cultured for 24 hours in blood agar plates were further inoculated in 10 mL of trypticase soy broth with 1% glucose and were incubated at 37°C for 24 hours. The individual wells of sterile 96 well-flat bottom polystyrene tissue culture plates (Sigma-Aldrich, USA) were filled with 200 μL of the diluted cultures (diluted 1 in 10 with fresh media). P. aeruginosa PAO1 strain was taken as positive control and also incubated, diluted and added to tissue culture plate. Sterile broth was used as negative control. After incubation at 37°C for 24 hours, gentle tapping was done. Washing with 0.2 mL of phosphate buffer saline (pH 7.2) four times was done to remove free floating ‘planktonic’ bacteria. Biofilm formed by bacteria adherent to the wells i.e., ‘sessile’ bacteria were fixed by 2% sodium acetate and stained by crystal violet (0.1%). Deionised water was used to remove excess stain and plates were kept for drying. Optical Density (OD) of the stained adherent bacteria was determined with a microplate reader at 590 nm wavelength [17] and calculated by comparing the OD of the negative control (ODc=0.068). The results were interpreted following the criteria of Stepanovic S et al., [18] as:
• Non-adherent | If ODisolates <ODc (0.068) |
• Weakly adherent | If ODc (0.068) ≤ODisolates <2x ODc (0.136) |
• Moderately adherent | If 2x ODc (0.136) ≤ODisolatse <4x ODc (0.272) |
• Strongly adherent | If 4x ODc (0.272) ≤ ODisolates |
Statistical Analysis
The socio-demographic data (age, gender, wards), number of collected samples, bacterial culture and their antimicrobial susceptibility pattern were taken manually from laboratory register and test results were analysed in counts and percentages using MS Excel 2010 version.
Results
Out of a total 3559 Gram negative bacilli isolated in two years, 826 (23.20%) were Non-fermenting Gram-Negative Bacilli (NFGNB). Among them 394 (47.69% of NFGNB) P. aeruginosa was identified from different clinical samples.
Demographic Distribution
Age and sex-wise distribution of 394 strains of P. aeruginosa is shown in the [Table/Fig-2]. Maximum number of cases (67.26%) was from adult populations (20-60 years) with mean age 39.9 years. Out of 394 strains of P. aeruginosa 288 (73.10%) were isolated from male patients whereas 106 (26.90%) were isolated from female patients.
Age-wise distribution of Pseudomonas aeruginosa isolates.
Age-group (years) | Number | Percentage (%) |
---|
Paediatric (0-12) | 69 | 17.51 |
Adolescent (13-19) | 15 | 3.81 |
Adult (20-60) | 265 | 67.26 |
Geriatric (>60) | 45 | 11.42 |
Total | 394 | 100 |
Resistance Pattern of P. aeruginosa Isolated from Clinical Samples
The resistance pattern showed 115 (29.18%) of P. aeruginosa were resistant to MRP followed by piperacillin/tazobactum 122 (30.96%), imipenem 132 (33.5%), aztreonam 137 (34.77%), cefoperazone-sulbactam 158 (40.1%), CAZ 181 (45.93%), gentamicin 203 (51.52%), levofloxacin 198 (50.25%) and ciprofloxacin 212 (53.80%) [Table/Fig-3]. Resistance to colistin were seen in 11 (2.79%) cases. Out of 394 isolates, 204 (51.77%) isolates of P. aeruginosa were found resistant to three or more antibiotic groups and considered as MDR P. Aeruginosa (MDR-PA). Moreover, 181 (45.93%) isolates of P. aeruginosa (n=394) resistant to CAZ were screened for ESBL and AmpC and 115 (29.18%) isolates resistant to MRP were screened for carbapenemase production [Table/Fig-4].
Antibiotic Resistance Pattern (%) of Pseudomonas aeruginosa.
TC: Ticarcillin-clavulanic acid; CAZ: Ceftazidime; CPM: Cefepime; CFS: Cefoperazone-sulbactam; PTZ: Piperacillin-tazobactam; IPM: Imipenem; MRP: Meropenem; DORI: Doripenem; AT: Aztreonam; CIP: Ciprofloxacin; LE: Levofloxacin; AK: Amikacin; GEN: Gentamycin; MINO: Minocycline; CL: Colistin
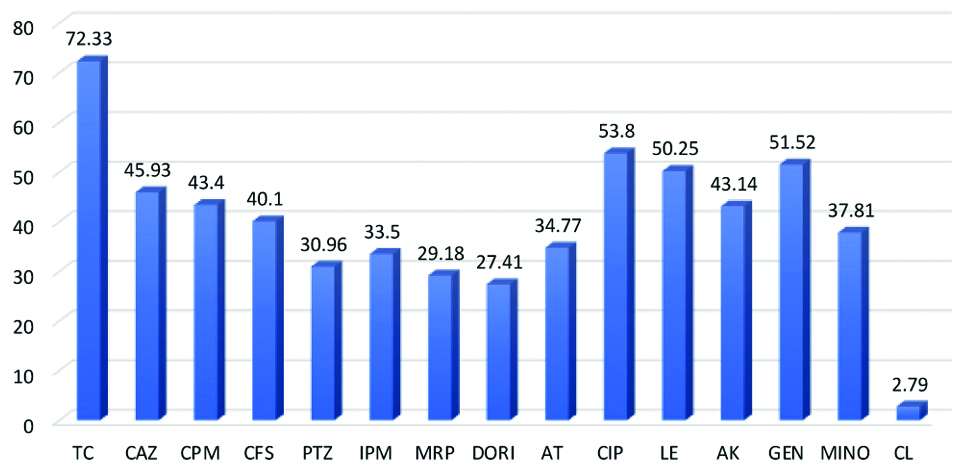
Sample wise distribution of Pseudomonas aeruginosa.
| Blood (Neonatal Septicaemia cases) | Blood and body fluids other than neonatal septicaemia cases | Urine | Pus/Wound swab | Sputum | ET | Central line tip | Total |
---|
Pseudomonas aeruginosa n (%) | 43 (10.92) | 79 (20.05) | 84 (21.32) | 117 (29.70) | 49 (12.43) | 13 (3.30) | 9 (2.28) | 394 (100) |
P.aeruginosa resistant to ceftazidime (for ESBL and AmpC screen) n (%) | 18 (41.86) | 31 (39.24) | 46 (54.76) | 61 (52.13) | 12 (24.48) | 8 (61.53) | 5 (55.56) | 181 (45.93) |
P.aeruginosa resistant to meropenem (for carbapenemase screen) n (%) | 10 (23.25) | 16 (20.25) | 30 (35.71) | 38 (32.47) | 14 (28.57) | 5 (38.46) | 2 (22.23) | 115 (29.18) |
ET: Endotracheal aspirate
Ward-wise distribution of P.aeruginosa isolates is shown in [Table/Fig-5]. Maximum isolates (20.05%) were from surgery ward followed by ITU/CCU/PICU/SNCU (15.99%) and chest ward (15.23%).
Ward-wise distribution of P.aeruginosa isolates.
Ward | No. (%) of bacteria isolated from indoor | No. (%) of bacteria isolated from out-patient department |
---|
Medicine | 41 (10.40) | 2 (0.51) |
ITU/CCU/PICU/SNCU | 63 (15.99) | -- |
Chest | 60 (15.23) | 2 (0.51) |
Surgery | 79 (20.05) | 1 (0.25) |
Orthopaedic | 29 (7.37) | 1 (0.25) |
ENT | -- | 14 (3.56) |
Urology | 43 (10.92) | 3 (0.76) |
Haematology | 26 (6.59) | -- |
Gynae and Obstetrics | 6 (1.52) | -- |
Paediatric | 23 (5.83) | 1 (0.25) |
Total | 370 (93.91) | 24 (6.09) |
ITU: Intensive treatment unit; CCU: Critical care unit; PICU: Paediatric intensive care unit; SNCU: Special newborn care unit; ENT: Ear nose and throat
Hospital and Community Acquired Infection
P. aeruginosa is a common pathogen of healthcare associated infections and most of the patients have underlying medical conditions like Chronic Obstructive Pulmonary Disease (COPD), cystic fibrosis etc., or risk factors like prolong Intensive Care Unit (ICU) stay, prior tracheostomy, on invasive devices or immunosuppression [2]. Although rare, Pseudomonas spp. may cause community acquired infections in immunocompetent individual. In this study, 370 (93.91%) infections were health-care associated and 24 (6.09%) were community acquired infections due to P. aeruginosa.
Phenotypic Detection of ESBL, MBL and AmpC Production by P. aeruginosa
In this study, ESBL (36.80%) was the most common β-lactamases produced by P. aeruginosa followed by AmpC (12.94%) and MBL (12.43%), respectively [Table/Fig-6].
Phenotypic detection of a) ESBL by CAZ/CAZ-CA (CAC), b) AmpC by CX/CXX, c) MBL by MRP/MRP-EDTA disk diffusion tests, respectively.
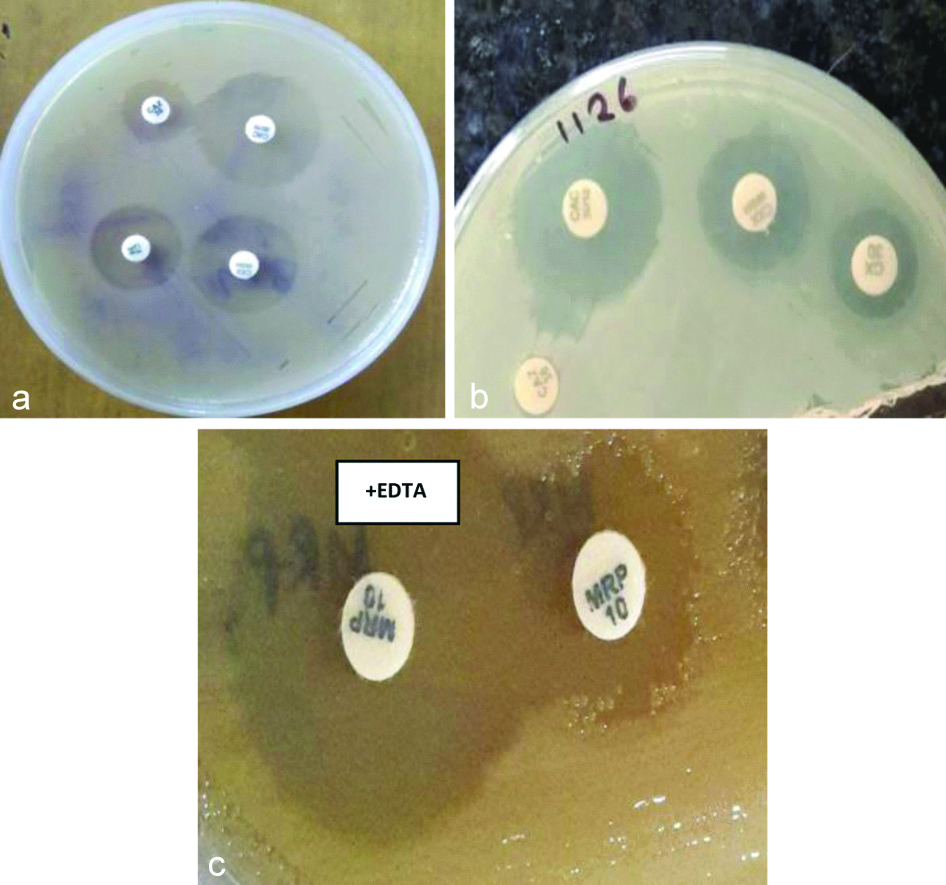
Single β-lactamase was produced by 113 (28.68%) isolates whereas multiple β-lactamase as co-production was seen in 64 (16.24%) isolates, i.e., production of 2 or 3 β-lactamases, simultaneously [Table/Fig-7].
Phenotypic detection of ESBL, MBL and AmpC production by P. aeruginosa.
Total β-lactamases (single +Co-production) producing isolates (%) among 394 P.aeruginosa isolates (n=394) | Single β-lactamase (%) | | Co-production of β-lactamases (%) |
---|
ESBL | 145 (36.80%) | 86 (21.83%) | | ESBL+MBL | 32 (8.12%) |
MBL | 49 (12.43%) | 8 (2.03%) | ESBL+AmpC | 23 (5.84%) |
AmpC | 51 (12.94%) | 19 (4.82%) | MBL+AmpC | 5 (1.27%) |
Total | 245 (62.18%) | 113 (28.68%) | ESBL+MBL+AmpC | 4 (1.01%) |
Total is not 394 because single Pseudomonas spp. in most cases were not only one carbapenemase producing but they were multiple carbapenemase producing strains
Detection of Carbapenemase Encoding Genes among Screened Isolates
Phenotypically by CDST, 49 (42.60%) isolates among 115 MRP resistant isolates were carbapenemase producing. Multiplex PCR was done in 30 representative strains (from the isolates which had similar antibiotic sensitivity, two/three representative isolates were taken) of MRP resistant Pseudomonas isolates from those 49 phenotypic carbapenemase producing isolates. blaNDM-1 (68.75%) was the most prevalent carbapenemases gene detected followed by blaVIM (18.75%) and co-production of blaNDM-1 + blaVIM was 12.5% [Table/Fig-8,9].
Genotypic distribution of MBL producing Pseudomonas aeruginosa.
Clinical samples | Meropenem resistant Pseudomonas (n=30) | Carbapenemase producing | VIM | NDM | Co-production of NDM+VIM |
---|
Blood from neonatal septicaemia cases | 8 | 4 (50%) | - | 4 (100%) | - |
Blood from adult septicaemia cases | 3* | 0 | - | - | - |
Urine | 10# | 7 (70%) | 1 (14.28%) | 6 (85.72%) | - |
Pus | 5@ | 3 (60%) | 2 (66.67%) | - | 1 (33.33%) |
Sputum and ET aspirate | 4 | 2 (50%) | - | 1 (50%) | 1 (50%) |
Total | 30 | 16 (53.34%) | 3 (18.75%) | 11 (68.75%) | 2 (12.5%) |
NDM: New Delhi metalo β-lactamase; VIM: Verona integron-encoded metallo-β-lactamase; IMP: Imipenemase
*Isolated from blood samples were carbapenem (MRP) resistant, but genotypically not carbapenemase producing
#Among these 10 Pseudomonas spp. 7 (70%) was genotypically carbapenemase producing and among these 7 isolates, 1 was VIM and 6 were NDM Positive{i.e. 6(NDM)+1(VIM)=7
@Among these 5 Pseudomonas spp., 3 were genotypically carbapenemase producing and out of these 3, 2 were VIM and 1 was NDM+VIM positive.{i.e. 2(VIM)+1(NDM+VIM)=3}
Multiplex PCR for the detection of carbapenemase producing genes in Pseudomonas aeruginosa isolates. Abbreviations: M, 100 bp DNA Ladder; Lane: 1,2,7,9,11,13 NDM positive bacteria (603 bp), Lane: 6 NDM+VIM positive bacteria, Lane: 10 VIM positive bacteria (437bp), Lane: 15 Positive control, Lane: 16 negative control.
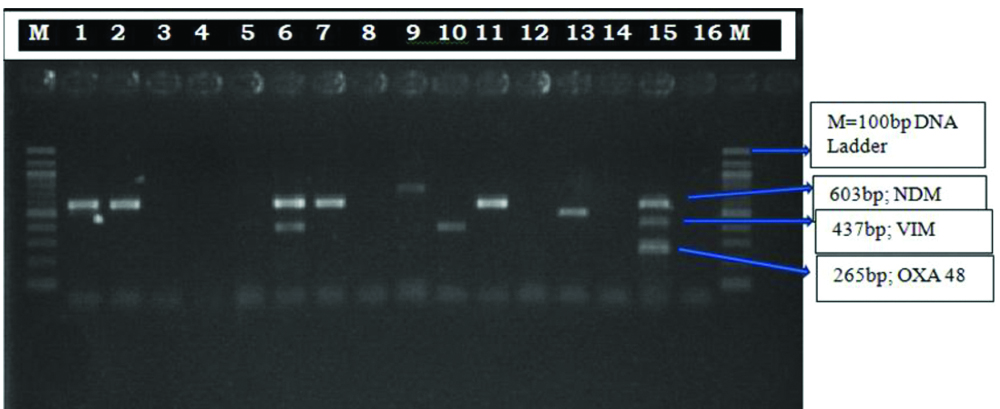
Biofilm Production
In P. aeruginosa infections biofilm production was studied as it is an important determinant of pathogenicity and responsible for antibiotic ineffectiveness. Tissue culture plate method was conducted as a quantitative assessment of biofilm production. The results have been shown that 158 (40.10%) isolates were biofilm producer. Among them, 78 were weak, 53 were moderate, and 27 were strong biofilm producer [Table/Fig-10]. To see whether there was any correlation between biofilm producing and antibiotic resistant strains, biofilm formation groups were analysed with respect to resistant phenotypes. Among the 27 strong biofilm-formers, 19 (70.37%) were non-MDR isolates and 8 (29.63%) were MDR ones and among 53 moderate biofilm-formers, 36 (67.92%) were non-MDR isolates and, 17 (32.08%) were MDR. The 78 weak biofilm-formers consisted of 26 (33.33%) non-MDR and 52 (66.67%) MDR isolates. So, non-MDR P.aeruginosa formed stronger biofilms than MDR-isolates and weak biofilms were formed more by MDR isolates than non-MDR ones. The 236 strains that were negative for biofilm formation consisted of 109 (46.19%) non-MDR and 127 (53.81%) MDR isolates.
Biofilm production by P.aeruginosa.
Total number of Pseudomonas isolates | Non-adherent (non-biofilm producer) | Biofilm producer 158 (40.10%) |
---|
Weakly adherent | Moderately adherent | Strongly adherent |
---|
394 | 236 (59.90%) | 78 (19.80%) | 53 (13.45%) | 27 (6.85%) |
Discussion
The prevalence of MDR-PA is alarmingly increasing throughout the world and the present study revealed the current scenario of drug resistance pattern with due emphasis on its β lactamase profile. In this study, 11.07% of P. aeruginosa was isolated among total 3559 Gram negative bacterial isolates, which was comparable with the isolation rate in various studies (14.38% by Kumari M et al.,) [19], (14.7% by Gill JS et al.,) [9]. The present study also showed 47.69% of Pseudomonas aeruginosa among Non-fermenter GNB (NF-GNB) which closely resemble with the study by Rahman M et al., [20] which showed 55.08% P. aeruginosa among NF-GNB. These data will help to predict local prevalence of P. aeruginosa infection.
Regarding demographic distribution, 265 (67.26%) P. aeruginosa were isolated from age group 20-60 years in present study with significant male preponderance and maximum 117 (29.70%) P. aeruginosa were isolated from pus and wound swab 117 (29.70%), followed by urine 84 (21.32%), similar with the study by Kumari M et al., which showed 22.57% and 20.90% of P. aeruginosa isolated from pus and urine samples, respectively [19].
In this study, the highest percentage (20.05%) of P. aeruginosa infections were observed in the surgical ward, followed by ITU/CCU/PICU/SNCU (15.99%) and chest ward (15.23%). Similar finding was observed in a study by Patel H and Garala RN which showed maximum P. aeruginosa were isolated from surgical ward (68%) [21]. Prevalence of infection was higher in surgical ward as maximum number of P.aeruginosa was isolated from wound/pus samples.
The antimicrobial resistance patterns observed in the present study revealed >50% isolates were resistant to fluroquinolones, gentamicin and ticarcillin-clavulanic acid. The treatment options for such strains are limited which may result in treatment failures and thereby causing significant morbidity and mortality. In this study the prevalence of MDR-PA was 51.77% whereas Gill JS et al., in their study found 50% and Prakash V et al., found 31.73% P. aeruginosa was MDR [9,22]. P. aeruginosa was intrinsically resistant to various β-lactam antibiotics and ertapenem in penem group because of its low outer membrane permeability [23,24], efflux system which expel antibiotic out of the bacterial cell [25,26] and production of antibiotic inactivating enzyme. Chromosomally mediated AmpC β-lactamase in P. aeruginosa confers natural resistance to lower level penicillins and cephalosporins [27]. In addition, the production of plasmid-mediated AmpC presents a new threat in the treatment of Pseudomonas spp. infections [4].
β-lactamases of Ambler Class A, C and D inactivate the β-lactams through the catalytic activity of serine-residue, whereas class B or MBLs need zinc for their action [28]. AmpC enzymes confer a high level of resistance to many β-lactam antibiotics including the third-generation cephalosporins and cephamycins (CX and cefotetan). The rates of ESBL and AmpC production in various clinical isolates of P. aeruginosa in this study was 36.80% and 12.94% whereas prevalence of ESBL was observed in a study by Easwaran S et al., was 57.75%, Dutta H et al., was 27.33% and Umadevi S et al., was 19.4% [29-31]. In a study by Upadhaya S et al., inducible AmpC was 7% [4]. In this study, the co-existence of AmpC and ESBL were observed in 23 in 394 isolates (5.84%) whereas Upadhyay S et al., reported in 4 out of 120 (3.34%) isolates and Easwaran S et al., reported the coexistence in 48 out of 70 isolates (68.57%) [4,29]. The treatment option for co-AmpC and ESBL producers are carbapenems. However, resistance to carbapenems has been increasing recently due to the production of MBL. Carbapenem resistant isolates in the present study was 29.18%. In India, the prevalence of MBLs ranges from 7.5 to 71 per cent [32]. Phenotypically, MBL production in present study was by 12.43% of all Pseudomonas isolates and 42.60% of carbapenem resistant isolates whereas by genotypic method 53.34% of carbapenem resistant Pseudomonas spp. were MBL producing. Rahman M et al., in their study showed 29.23% P.aeruginosa were resistant to carbapenem and all were NDM producing [20].
Biofilm formation is one of the additional antibiotic resistance mechanisms in P. aeruginosa. They exhibit antibiotic resistance by restricted penetration of antibiotics into biofilm mass, decreased growth rate, and expression of resistant genes [33]. The bacteria can produce biofilm on the hospital surfaces and catheters or any device, cause therapeutic complications and consequently increased length of stay in hospital, which finally impose increased costs for therapy [34]. In this study, 40.10% of isolates were biofilm producer whereas in a study by Kádár B et al., 23.3% of P. aeruginosa were biofilm producer [35]. In another study by Ghadaksaz A et al., in Iran, the frequency of biofilm production was 47.1% [36]. In a study conducted at Manipal, 68% of P. aeruginosa strains were associated with biofilm production [37]. According to this study, MDR P.aeruginosa did not show a trend to form stronger biofilms than non-MDR isolates which might imply that, bacteria, to get a better survival, formed stronger biofilm in isolates with low level of resistance and formed weak biofilm by isolates with high level of resistance to achieve similar level of protection. Abidi SH et al., concluded that biofilm production was significantly higher in MDR Pseudomonas spp. isolates [38], whereas Cepas V et al., failed to find a significant difference in biofilm formation among MDR and non-MDR isolates [39].
P. aeruginosa mostly causes health care associated infections with low likelihood of community acquired infections. The prevalence of MDR Pseudomonas spp. according to this study was more than 50%. Pan India susceptibility profile of P. aeruginosa varies from one region to another with the prevalence of different antibiotic resistant genes [40]. Resistance to antibiotics limits therapeutic options and leads to increased mortality and morbidity. Apart from that, high propensity to form biofilm by Pseudomonas spp. will add to its virulence and antibiotic resistance. This study helps to detect the local prevalence, drug resistant pattern and the mechanism of drug resistance of P.aeruginosa.
Limitation(s)
Genes encoding ESBL and AmpC production could not be detected due to limited resources.
Conclusion(s)
The management of infections caused by MDR P. aeruginosa is a major challenge to the clinicians because such strains offer resistance to most of the routinely used antibiotics. Early detection of these β-lactamase production is crucial for the initiation of appropriate antibiotic therapy and infection control policy. Continuous surveillance of P. aeruginosa strains in the hospital settings as well as in the communities is therefore of vital importance to manage the infections effectively. Furthermore, strict antibiotic policies have to be implemented to limit the irrational use of antibiotics in the hospital environment.