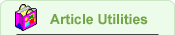
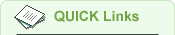
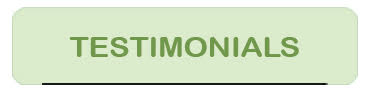
Authors are the souls of any journal, and deserve much respect. To publish a journal manuscripts are needed from authors. Authors have a great responsibility for producing facts of their work in terms of number and results truthfully and an individual honesty is expected from authors in this regards. Both ways its true "No authors-No manuscripts-No journals" and "No journals–No manuscripts–No authors". Reviewing a manuscript is also a very responsible and important task of any peer-reviewed journal and to be taken seriously. It needs knowledge on the subject, sincerity, honesty and determination. Although the process of reviewing a manuscript is a time consuming task butit is expected to give one's best remarks within the time frame of the journal.
Salient features of the JCDR: It is a biomedical, multidisciplinary (including all medical and dental specialities), e-journal, with wide scope and extensive author support. At the same time, a free text of manuscript is available in HTML and PDF format. There is fast growing authorship and readership with JCDR as this can be judged by the number of articles published in it i e; in Feb 2007 of its first issue, it contained 5 articles only, and now in its recent volume published in April 2011, it contained 67 manuscripts. This e-journal is fulfilling the commitments and objectives sincerely, (as stated by Editor-in-chief in his preface to first edition) i e; to encourage physicians through the internet, especially from the developing countries who witness a spectrum of disease and acquire a wealth of knowledge to publish their experiences to benefit the medical community in patients care. I also feel that many of us have work of substance, newer ideas, adequate clinical materials but poor in medical writing and hesitation to submit the work and need help. JCDR provides authors help in this regards.
Timely publication of journal: Publication of manuscripts and bringing out the issue in time is one of the positive aspects of JCDR and is possible with strong support team in terms of peer reviewers, proof reading, language check, computer operators, etc. This is one of the great reasons for authors to submit their work with JCDR. Another best part of JCDR is "Online first Publications" facilities available for the authors. This facility not only provides the prompt publications of the manuscripts but at the same time also early availability of the manuscripts for the readers.
Indexation and online availability: Indexation transforms the journal in some sense from its local ownership to the worldwide professional community and to the public.JCDR is indexed with Embase & EMbiology, Google Scholar, Index Copernicus, Chemical Abstracts Service, Journal seek Database, Indian Science Abstracts, to name few of them. Manuscriptspublished in JCDR are available on major search engines ie; google, yahoo, msn.
In the era of fast growing newer technologies, and in computer and internet friendly environment the manuscripts preparation, submission, review, revision, etc and all can be done and checked with a click from all corer of the world, at any time. Of course there is always a scope for improvement in every field and none is perfect. To progress, one needs to identify the areas of one's weakness and to strengthen them.
It is well said that "happy beginning is half done" and it fits perfectly with JCDR. It has grown considerably and I feel it has already grown up from its infancy to adolescence, achieving the status of standard online e-journal form Indian continent since its inception in Feb 2007. This had been made possible due to the efforts and the hard work put in it. The way the JCDR is improving with every new volume, with good quality original manuscripts, makes it a quality journal for readers. I must thank and congratulate Dr Hemant Jain, Editor-in-Chief JCDR and his team for their sincere efforts, dedication, and determination for making JCDR a fast growing journal.
Every one of us: authors, reviewers, editors, and publisher are responsible for enhancing the stature of the journal. I wish for a great success for JCDR."
Thanking you
With sincere regards
Dr. Rajendra Kumar Ghritlaharey, M.S., M. Ch., FAIS
Associate Professor,
Department of Paediatric Surgery, Gandhi Medical College & Associated
Kamla Nehru & Hamidia Hospitals Bhopal, Madhya Pradesh 462 001 (India)
E-mail: drrajendrak1@rediffmail.com
On May 11,2011
Dr. Shankar P.R.
"On looking back through my Gmail archives after being requested by the journal to write a short editorial about my experiences of publishing with the Journal of Clinical and Diagnostic Research (JCDR), I came across an e-mail from Dr. Hemant Jain, Editor, in March 2007, which introduced the new electronic journal. The main features of the journal which were outlined in the e-mail were extensive author support, cash rewards, the peer review process, and other salient features of the journal.
Over a span of over four years, we (I and my colleagues) have published around 25 articles in the journal. In this editorial, I plan to briefly discuss my experiences of publishing with JCDR and the strengths of the journal and to finally address the areas for improvement.
My experiences of publishing with JCDR: Overall, my experiences of publishing withJCDR have been positive. The best point about the journal is that it responds to queries from the author. This may seem to be simple and not too much to ask for, but unfortunately, many journals in the subcontinent and from many developing countries do not respond or they respond with a long delay to the queries from the authors 1. The reasons could be many, including lack of optimal secretarial and other support. Another problem with many journals is the slowness of the review process. Editorial processing and peer review can take anywhere between a year to two years with some journals. Also, some journals do not keep the contributors informed about the progress of the review process. Due to the long review process, the articles can lose their relevance and topicality. A major benefit with JCDR is the timeliness and promptness of its response. In Dr Jain's e-mail which was sent to me in 2007, before the introduction of the Pre-publishing system, he had stated that he had received my submission and that he would get back to me within seven days and he did!
Most of the manuscripts are published within 3 to 4 months of their submission if they are found to be suitable after the review process. JCDR is published bimonthly and the accepted articles were usually published in the next issue. Recently, due to the increased volume of the submissions, the review process has become slower and it ?? Section can take from 4 to 6 months for the articles to be reviewed. The journal has an extensive author support system and it has recently introduced a paid expedited review process. The journal also mentions the average time for processing the manuscript under different submission systems - regular submission and expedited review.
Strengths of the journal: The journal has an online first facility in which the accepted manuscripts may be published on the website before being included in a regular issue of the journal. This cuts down the time between their acceptance and the publication. The journal is indexed in many databases, though not in PubMed. The editorial board should now take steps to index the journal in PubMed. The journal has a system of notifying readers through e-mail when a new issue is released. Also, the articles are available in both the HTML and the PDF formats. I especially like the new and colorful page format of the journal. Also, the access statistics of the articles are available. The prepublication and the manuscript tracking system are also helpful for the authors.
Areas for improvement: In certain cases, I felt that the peer review process of the manuscripts was not up to international standards and that it should be strengthened. Also, the number of manuscripts in an issue is high and it may be difficult for readers to go through all of them. The journal can consider tightening of the peer review process and increasing the quality standards for the acceptance of the manuscripts. I faced occasional problems with the online manuscript submission (Pre-publishing) system, which have to be addressed.
Overall, the publishing process with JCDR has been smooth, quick and relatively hassle free and I can recommend other authors to consider the journal as an outlet for their work."
Dr. P. Ravi Shankar
KIST Medical College, P.O. Box 14142, Kathmandu, Nepal.
E-mail: ravi.dr.shankar@gmail.com
On April 2011 Anuradha
Dear team JCDR, I would like to thank you for the very professional and polite service provided by everyone at JCDR. While i have been in the field of writing and editing for sometime, this has been my first attempt in publishing a scientific paper.Thank you for hand-holding me through the process.
Dr. Anuradha
E-mail: anuradha2nittur@gmail.com
On Jan 2020
JCDR is now Monthly and more widely Indexed .
- Emerging Sources Citation Index (Web of Science, thomsonreuters)
- Index Copernicus ICV 2017: 134.54
- Academic Search Complete Database
- Directory of Open Access Journals (DOAJ)
- Embase
- EBSCOhost
- Google Scholar
- HINARI Access to Research in Health Programme
- Indian Science Abstracts (ISA)
- Journal seek Database
- Popline (reproductive health literature)
- www.omnimedicalsearch.com